TP53: A unique tumor suppressor
Tumor suppressor proteins play a critical role in preventing cells from becoming damaged and malignant. In this review, we discuss the discovery of the tumor suppressor gene TP53 and delve into the cellular pathways that TP53 can induce to elicit its function as a tumor suppressor. Mutations in TP53 frequently occur in cancer cells and lead to the expression of a stabilized mutated protein, a feature that is unique for a tumor suppressor protein. We discuss the effect of mutant TP53 on cancer growth and the implications for cancer therapy.
Schlüsselwörter: TP53, tumorigenesis, tumor suppressor gene
As we age, our DNA naturally acquires changes, or mutations, due to a mixture of environmental factors and internal cellular errors. Some mutations are inconsequential; however, some are much more detrimental and can cause diseases such as cancer. As our life expectancy increases, our cells have more time to gain these mutations, and this increases the prevalence of these diseases in our population.
Our bodies have inherent mechanisms to detect damaged cells and thereby prevent unhealthy cells from dividing. One such mechanism is through so-called “tumor suppressor proteins” that regulate cell growth and replication by performing checks on the cells as they go through the cell cycle. Damaged cells can have their DNA repaired, or in some cases, the unhealthy cell can be triggered to commit cell suicide. One of the most well-known tumor suppressor proteins is TP53.
In this review, we will discuss the discovery of TP53 and its role in preventing cancer. We will also focus on the role of mutant TP53 in cancer, an aspect that is unique for a tumor suppressor protein.
Discovery of tumor suppressor genes
Until the late 1970s, tumor suppressor genes (TSGs) were considered to be an unproven hypothesis. Knudson used the two-hit hypothesis to explain the hereditary nature of the Retinoblastoma protein (RB) in 1971 [1] (the theory that both copies of the gene need to be inactive to cause disease). However, it took until 1986 for this gene to be recognized as a TSG [2]. Throughout the 70s and early 80s, oncogenes became the prevailing theory as to the molecular cause of cancer, with more and more of these tumor activating genes, such as MYC, RAS, and SRC, being described. It was not until 1985 that the term “tumor suppressor gene” was used for the first time, and not until the early 90s when it became common practice to use TSG instead of the more commonly used term “anti-oncogene”.
TP53 was the second TSG to be named after RB. However, the pathway to discovery was complex for TP53 because it was initially reported in 1979 to be an oncogene by six independent groups [3] (Fig. 1).
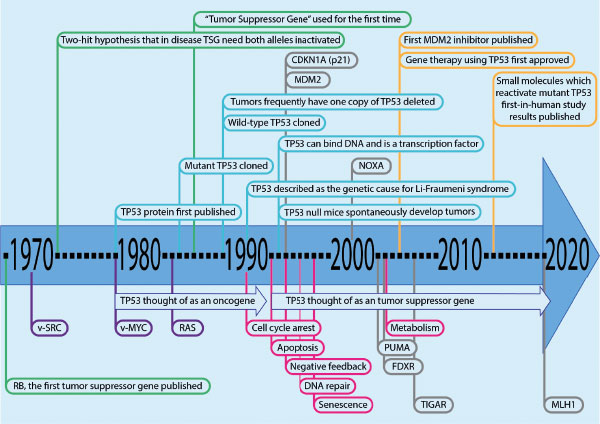
Work from five of these groups used the simian virus, SV40, to transform cells, and found a ~ 50 kDa protein to be bound to the large T antigen produced by this virus. The sixth group saw a protein of the same size in a mouse sarcoma model.
To uncover the function of TP53, early researchers cloned the cDNA encoding the TP53 protein and expressed it in cells. Expression of this cloned TP53 enhanced cell proliferation and cell survival and was able to transform cells to become malignant. It was also observed that there was an accumulation of the TP53 protein in cancer cells. Therefore, TP53 was initially reported to be an oncogene, and this became the dogma for the subsequent 10 years.
However, in the late 80s, it was shown that the initially cloned sequences of TP53 were, in fact, mutant forms of the protein [3], and that human colorectal cancers with these protein accumulations often had a chromosomal deletion that removed the wild-type (wt) copy of the TP53 gene [4]. From this point, it became a priority to investigate the actual function of wt TP53.
TP53’s role in preventing cancer
Wt TP53 turned out to be a different beast entirely. Over-expressing the wt TP53 gene was disadvantageous to cell survival, and in rat fibroblasts, wt TP53 could not transform these cells like mutant TP53 could [5]. The consensus that it was a TSG came after Trp53 (mouse version of TP53) gene knockout mice were shown to develop spontaneous tumors with 100 % penetrance in under a year [6]. Additionally, patients with Li-Fraumeni syndrome were prone to spontaneous tumor development and were found to have only one copy of the TP53 gene [7].
TP53 was found to be a transcription factor with a bona fide DNA binding domain and a recognition sequence for TP53 on DNA [3]. TP53 acts as a tumor suppressor through the activation of multiple processes associated with slowing down cell growth, including the induction of cell cycle arrest, senescence, apoptosis, DNA repair and metabolism changes.
Cell cycle arrest was the first described function of wt TP53. Expression of wt TP53 in rat fibroblasts induced growth arrest [5]. This was also shown using temperature-sensitive mutants of TP53, where at 37.5 °C, a mutated protein would be expressed that could transform the growth of cells. However, at 32.5 °C, the activity of the mutant TP53 is suppressed, wt TP53 protein would be expressed, and cells would undergo growth arrest [8]. TP53 has since been shown to upregulate a myriad of other important cell cycle regulatory genes, including P21, encoded by CDKN1A, which controls the G1/S cell cycle transition [9].
TP53 has been implicated in the induction of both intrinsic and extrinsic apoptosis. Again using temperature-sensitive mutants, expression of wt TP53 induced apoptosis in acute myeloid leukemia derived cell lines [10]. Studies have now shown that TP53 mediated intrinsic apoptosis is initiated by upregulation of the BH3-only protein and direct TP53 target, PUMA, with the BH3-only protein NOXA playing a secondary role [11]. In the extrinsic apoptosis pathway, DR5 and FAS are TP53 direct target genes, but they are not critical for TP53-mediated apoptosis.
The role of TP53 in DNA repair has been complex to unravel. Studies have indicated that functioning TP53 was critical for DNA repair after UV damage [12], yet non-essential in preventing irradiation-induced thymomas [13]. Recently, in studies in lymphoma, the TP53 target gene Mlh1 was shown to be crucial for tumor prevention [14]. This conflicting data could indicate that the DNA repair function of TP53 is context-specific and depends on the particular repair pathway activated, a finding that warrants further investigation.
Evidence is emerging that TP53 can exert many changes in the metabolic state of the cell. Several genes involved in metabolism are direct targets of TP53, including TIGAR, which inhibit glycolysis, and FDXR, which functions to enhance oxidative phosphorylation [9]. TP53 also indirectly regulates many other components of metabolic pathways that are repressed in response to TP53 activation.
The observations that wt TP53 can play a critical role in so many different cellular processes may explain why TP53 is such a powerful TSG and likely explains why TP53 or the TP53 pathway is found to be mutated and inactivated in a large proportion of human cancers.
TP53 in established cancers
TP53 plays a fundamental role in preventing cancer. However, controversially for a TSG, once TP53 is mutated, it is postulated to promote tumor growth.
The mutational spectrum of TP53 found in cancers is unique for a TSG. The mutations are most commonly single nucleotide changes found in the DNA binding domain (~ 95 %) that cause a single amino acid substitution and allows the protein to stabilize and accumulate in the cell, instead of being degraded [15]. These mutated TP53 proteins have lost the ability to bind to DNA and so cannot transcriptionally upregulate wt TP53 target genes. In line with the two-hit hypothesis, that both copies of a TSG need to be disabled to cause cancer, there is frequent (> 90 %) loss of the remaining wt TP53 allele [15], leaving only the highly expressed mutant protein present within the cell. This explains why the initial studies into TP53 function that were conducted in cancer cells, concluded that TP53 was an oncogene because they were studying mutated forms of the protein.
The mutant TP53 protein is thought to affect cancer growth in three different ways: loss-of-function, dominant-negative effects on the wt TP53 protein (if present) and neomorphic gain-of-function activities that promote tumor growth (Fig. 2).
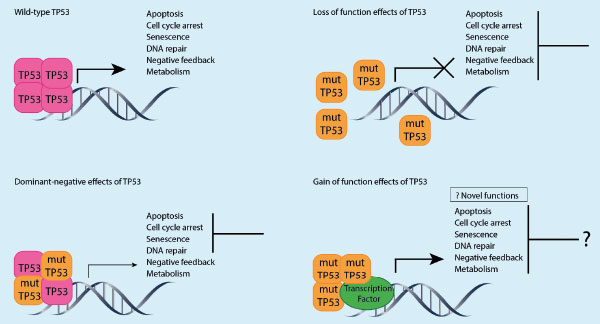
Firstly, mutations in TP53 lead to loss-of-function of wt tumor suppressor processes because the mutant TP53 cannot activate expression of the TP53 target genes that induce cellular responses. These loss-of-function mutations can be modeled using Trp53 null mice, which develop spontaneous tumors, mostly lymphomas, within the first year of life [6].
Mutant TP53 can also play a role in tumor development through dominant-negative effects (DNEs) on the co-expressed wt TP53 protein. The TP53-mediated DNEs appear complex because it is reported that mutant TP53 can preferentially allow certain tumor advantageous activities of the wt TP53 protein that could contribute to cell growth and proliferation, and yet suppress other tumor suppressor functions such as apoptosis [16, 17]. The underlying mechanisms of how this selectivity arises are still under investigation. Nevertheless, DNEs represent a crucial early step in tumorigenesis. Once a tumor is established, loss of the wt allele frequently occurs. At this stage, the DNE of mutant TP53 no longer has a role in tumorigenesis, and putative gain-of-function (GOF) effects are likely to become more critical.
The GOF activity of mutant TP53 is where the mutated protein has activities outside of the normal functions of wt TP53 that can contribute to tumor growth. Evidence for GOF activities of mutant TP53 has come from mouse models where the expression of a mutant TP53 protein has resulted in different tumor spectrums and increased metastatic potential, compared to Trp53 null mice [6]. It is thought that the mutant TP53 protein can interact with other transcription factors, or chromatin-remodeling complexes [18], activating their downstream target genes and inducing these tumor-promoting GOF activities.
Whether the GOF effects of mutant TP53 are essential for the sustained growth of the established tumor, has important implications for cancer therapy. Studies have shown that the withdrawal of mutant TP53 from established tumors in vivo results in tumor regression, indicating the tumor cells rely on mutant TP53 expression for continued tumorigenic potential [19]. This would indicate that mutant TP53 itself could be a therapeutic target. However, this is controversial as another study has indicated that the removal of mutant TP53 from established cancer cell lines has little impact on the growth of the tumor cells since this leads to a TP53 null state [20]. This would suggest that research efforts should focus on designing drugs that induce the activity of potent tumor suppressor proteins downstream of wt TP53 since these drugs would have the most potential for cancer therapy.
Conclusions and further questions
Although there have been 40 years of research into TP53, many outstanding questions still need to be addressed, in particular, which TP53 target genes are critical for its tumor-suppressive function. There is not yet a consensus regarding which genes are direct targets of TP53 and whether TP53 can also function as a transcriptional repressor as well as a transcriptional activator. Meta-analysis of ChIP binding data and RNA sequencing data [9] are providing essential insights into these questions by slowly narrowing this list down to direct and indirect targets.
Another outstanding question is whether one particular cellular pathway regulated by TP53 is the most important for tumor suppression or conversely, whether, TP53 is an effective TSG because it can regulate a wide breadth of target genes from multiple effector pathways. If the latter option holds, then how do all these pathways interact with each other to induce effective tumor suppression, and why do different cell types respond with one predominant outcome, such as growth arrest or apoptosis, following TP53 activation. Understanding these fundamental questions could enable us to manipulate the response of cancer cells to current therapies or design novel drugs that could better harness TP53’s control over the cell.
Furthermore, it is not yet clear if mutant TP53 itself represents a promising therapeutic target since the postulated GOF activities of mutant TP53 are still under investigation. However, an understanding of the wt TP53 targets that can induce tumor suppression would enable the design of novel drugs that could directly induce the expression of these genes, bypassing mutant TP53. Significant efforts and resources are being invested in addressing these outstanding issues.
TP53 represents just one TSG present within our cells, and since the 1980s, we have uncovered a plethora of others. However, challenges remain in identifying new TSGs. We need to develop more efficient and accurate methodologies to identify TSGs, and to demonstrate that the loss/inactivation of a candidate gene drives cancer formation or allows uncontrolled tumor growth. This will aid us in understanding the vast heterogeneity of cancer cells and provide opportunities to improve therapeutic options in the longer term.