Establishment of a DFG-funded research group on the topic of plant-microbe communication through extracellular RNA
DOI: https://doi.org/10.47184/tev.2021.01.02The exRNA consortium, a team of researchers funded by the Deutsche Forschungsgemeinschaft (DFG), addresses crucial aspects of cross-kingdom RNA interference (ckRNAi) and RNA application in plant protection, mainly focusing on mechanistic considerations and application efficiencies.
Keywords: Extracellular RNA, exosomes, double-stranded RNA
Introduction
According to the scientific literature, there is no other option than to increase the global yield efficiency and reduce the yield gap to guarantee future global food security – given that further land increase for agriculture is not an acceptable alternative (EPRS 2019). To realize these agricultural goals a new understanding of the mechanisms underlying plant diseases/immunity and new technological developments in crop protection are required. Recent knowledge suggests that agricultural pests and pathogens can be controlled by exogenous or transgene-mediated supply of double-stranded (ds)RNA targeting essential microbial/pest genes: plants expressing target-specific dsRNAs are more resistant to viroids, viruses, bacteria, fungi, oomycetes, nematodes, and insects (for a topical overview see Cai et al. 2018a; Cai et al. 2019; Liu et al. 2020; Šečić et al. 2021).
The broad applicability of engineered RNA interference (RNAi) techniques is in good agreement with recent findings of small (s)RNA trafficking between interacting organisms. In fact, first hints for natural cross-kingdom RNAi (ckRNAi), and the action of fungal sRNA effectors in plants came from studies on plant (barley) transgene-mediated delivery of dsRNA into the pathogenic powdery mildew fungus (Nowara et al. 2010). Subsequently, exchange of sRNAs between the model plant Arabidopsis thaliana and the pathogenic grey mold fungus Botrytis cinerea was demonstrated (Weiberg et al. 2013); and such sRNA exchange turned out to be bidirectional (Wang 2016, Zhang 2016, Cai 2018b). There is accumulating evidence from other plant-pathogen systems across kingdoms (fungi, oomycetes, bacteria) that similarly ckRNAi may modulate the respective interactions, pointing to conservation of the underlying principle. Thus, understanding the basic mechanisms of ckRNAi in diverse plant-microbe interactions will increase our knowledge in plant-microbe RNA comunication and help to improve the agronomic application of dsRNA. Of note, recent reports have challenged the generalization of the ckRNAi model because not all pathogens/pests seem to be amenable to exogenous RNA (Kogel 2021; Qiao et al. 2021; Šečić and Kogel 2021) or/and lack critical components of an RNAi pathway (Nicolás et al. 2013; Kettles et al. 2018; Šečić and Kogel 2021).
An RNAi-based technology has already been approved by the Canadian Food Inspection Agency in 2016 and the US Environmental Protection Agency in 2017 (Head et al. 2017), indicating its market validity. However, while numerous reports provide proof-of-concept towards RNA applications in crop protection, many questions remain unsolved regarding scientific, regulatory and safety issues (Kookana et al. 2014). Although GMO (genetically modified organism) strategies are already approved, alternative technologies such as direct dsRNA delivery have been suggested and may have a better prognosis for application because of the higher public acceptance of non-GMO techniques. The latter technology has some technical advantages such as flexibility of target selection and high potential for immediate adaptation to emerging pests and diseases. While the exRNA consortium is clearly focused on plant-microbe interactions, it is important not to ignore the knowledge about “pest” control by RNA as it is more advanced and mechanistic aspects, albeit quite different from microbes, are better understood (Figure 1).
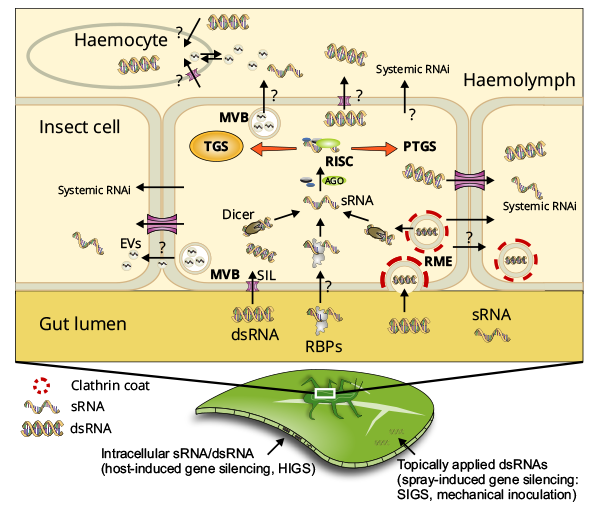
Figure 1: RNA-based plant protection against insects. Ingestion of dsRNA/sRNA present on the plant surface and/or inner tissues by an insect predator leads to their presence in the insect gut lumen. Uptake from the insect gut appears to be accomplished primarily by clathrin-dependent receptor-mediated endocytosis (RME), which preferentially imports long dsRNA over sRNA; additionally, SID1-like (SIL)-proteins may play a role. Studies of mammalian and plant-microbe systems suggest that dsRNA/sRNA uptake also might occur via extracellular vesicles (EVs). RNA-binding proteins (RBPs) may support dsRNA/sRNA transfer, although their role is not well understood. For many insects, uptake of long dsRNA induces RNAi more efficiently than short sRNA. However, since HIGS is triggered by in planta expression of dsRNA, which is largely processed into sRNA by plant DICER-like (DCL) proteins, the size requirement may be variable and/or a sRNA uptake mechanism(s) may exist (from Liu et al. 2019).
The key objectives of the consortium are listed in Box 1.
Box 1: Key objectives of the exRNA consortium
Elucidating the abundance and principles of ckRNAi in plant-microbe interactions
It is unclear how widespread ckRNAi is in nature (Ma et al. 2020). In 2013, the group of Hailing Jin, Riverside (USA), discovered sRNAs of Botrytis cinerea that invade host cells during infection and hijack the plant RNAi pathway to silence important immunity genes (Weiberg et al. 2013). Since then, sRNA-mediated plant gene silencing has also been proposed for the fungal pathogens Puccinia striiformis, Blumeria graminis and Magnaporthe oryzae (Wang et al. 2017; Kusch et al. 2018; Zanini et al. 2019) and was found in the oomycete pathogen Hyaloperonospora arabidopsidis (Dunker et al. 2020) and during beneficial rhizobial infection and nodule formation in legumes (Ren et al. 2019). Plant RNAs also translocate into fungal pathogens during infection: Verticillium dahliae cells recovered from infected cotton accumulate plant micro (mi)RNAs.
Two of these cotton miRNAs target fungal pathogenicity genes (Zhang et al. 2016). Moreover, Arabidopsis shuttles defensive sRNAs into Botrytis cinerea (Cai et al. 2018b).
Yet, it is an open question whether the ckRNAi model is generally applicable (as one would argue for protein effectors) and whether it applies to agronomically important mutualistic plant-fungus systems such as mycorrhizae and endophytes. There are also examples of RNA exchange in animal-parasite interactions. Sickle-cell erythrocytes, which are resistant to the malaria parasite Plasmodium falciparum, transfer miRNAs and block parasite mRNA translation (LaMonte et al. 2012). Finally, mouse and human gut-derived miRNAs can be taken up by E. coli and Fusobacterium nucleatum to regulate bacterial transcript levels affecting growth (Liu et al. 2016). Hence, exchange of RNAs between interacting organisms seems to be widespread in nature; however, different mechanistic principles might be involved: RNA communication is not restricted to small interfering (si)RNA and miRNA, defined in sensu stricto as 20 to 24 nts long duplexes, which are the trigger for canonical RNAi (Baulcombe 2015), but also include tRNA or tRNA fragments. An example is the above-mentioned Rhizobium-legume interaction (Ren et al. 2019). Moreover, the protozoan parasite Trypanosoma cruzi secretes tRNA-derived sRNAs, which contribute to infecting mammalian cells. P. falciparum and T. cruzi both have lost canonical RNAi factors of DICER (DCL) and ARGONAUTE (AGO) proteins (Garcia-Silva et al. 2014), suggesting host and parasite sRNAs trigger RNAi-independent gene expression modulation.
Assessing the routes of RNAs in plant-microbe ckRNAi
The underlying mechanisms and functions of RNA transport between plants and microbes are largely unknown (Cai et al. 2021). In mammals, intercellular communication is partly mediated by circulating RNAs, which are transported with the support of distinct types of extracellular vesicles (EVs), specific transmembrane proteins, high-density lipoprotein complexes, and gap junctions (Mittelbrunn et al. 2011). The parasitic nematode Heligmosomoides polygyrus produces EVs during infection that seem to hijack the host EV-dependent cell-to-cell communication infrastructure to deliver parasitic sRNAs into host cells (Buck et al. 2014).
In plants, sRNAs can move between adjacent cells, presumably through plasmodesmata, while systemic transport of diverse RNAs appears to occur through the phloem (Molnar et al. 2010; Kehr and Kragler 2018). Moreover, plants secrete RNA-containing EVs into the extracellular space (apoplast) (Cai et al. 2018b; Baldrich et al. 2019). In plant-fungal interactions, RNAi-inducing regulatory sRNAs translocate from plant cells into hyphae, a phenomenon called host-induced gene silencing (HIGS, Nowara et al. 2010; Koch et al. 2013). In Arabidopsis thaliana, EV-sRNAs are delivered into fungal cells during infection to suppress virulence genes (Cai et al. 2018b), proposing EVs as a plausible route of RNA transport from plants to pathogens (Figure 2).
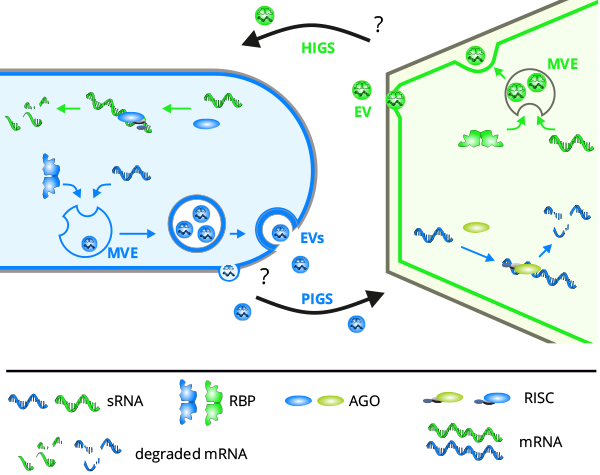
Figure 2: ckRNAi at the fungus-plant interface mediated by EVs. During infection, both the fungus and the plant deploy sRNAs to silence target genes in the interaction partner, as virulence and defense strategies, respectively. Silencing of fungal genes by host sRNAs is termed HIGS and vice versa, pathogen-induced gene silencing (PIGS) is brought about by fungal sRNAs in plants. EVs are one of the ways how sRNAs are transferred. EVs can be derived from multivesicular endosomes (MVEs, syn multivesicular bodies, MVBs) or from budding at the plasma membrane (PM). Endosomes bud inwards during maturation to form intraluminal vesicles, incorporating contents from the cytosol, notably sRNAs and proteins. RBPs are thought to be key determinants of RNA loading into EVs. Intraluminal vesicles are released as exosomes upon fusion of the MVE with the PM. How precisely EVs cross the cell walls and deliver their contents to the recipient cell are currently undetermined (from Kwon et al. 2019).
Remarkably, sRNA loading into EVs is likely to be a selective process in plants and animals (Koppers-Lalic et al. 2014, Preußer et al. 2018; Baldrich et al. 2019), suggesting a supportive role of RNA-interacting factors, such as RNA-binding proteins (RBPs), in EV-RNA mediated cell-to-cell communication. Of note, recent work suggests EV-independent transfer of sRNA between host plants and fungi (Baldrich et al. 2019). Importantly, all above assumptions are based on in vitro analysis of more or less purified EV-like particles, but we do not unequivocally know the biological function of EVs, also not for RNA transport, showing the need to develop tools to research EVs in action.
Discovering the key transacting factors involved in the transport of RNAs
A central hypothesis in the exRNA consortium is that for the mechanisms of sRNA transport similar routes as known from mRNA transport are also operational (Mofatteh et al. 2017). In cells, RNAs typically form higher-order ribonucleoprotein (RNP) complexes and intercellular RNA transport is likely based on the formation of RNP complexes (He et al. 2021). This concept was studied for intracellular long-distance transport of mRNAs alongside microtubules in Ustilago maydis. The key RBP Rrm4, in close association with the accessory RBP Grp1, transports thousands of mRNAs throughout the growing infectious hyphae (Haag et al. 2015; Olgeiser et al. 2019). This takes place at the cytoplasmic surface of endosomes, thereby linking RNA and membrane trafficking, an emerging field in RNA biology (Béthune et al. 2019; Kwon et al. 2019). Noteworthy, AGO proteins and sRNAs forming the functional RNA-induced silencing complex (RISC) are also proposed to be linked to endomembrane compartments in plants and animals (e. g. Kim et al. 2014). Moreover, human AGO2 together with GW182 is associated with endosomes and multivesicular body (MVB) markers, and EV proteome analysis in the nematode H. polygyrus identified a worm-specific AGO, exWAGO (Chow et al. 2019), proposing that AGOs interact with highly conserved secretory pathway components that exist in fungi, plants, and animals. However, bacteria have no canonical AGO and they do not release EVs from MVBs but instead through blebbing or cell lysis, indicating major differences between pro- and eukaryotes in these processes. Beyond AGOs, other proteins have been reported to participate in sRNA loading into EVs in animals (Shurtleff et al. 2017; Santangelo et al. 2016), and other RBPs that specifically recognize sRNAs like the plant protein CmPSRP1 (Kehr & Kragler 2018) or dsRNA-specific transporters could be involved in RNA export or import. A critical question is how specificity is achieved in a way that distinct RNAs are packaged into EVs for transport. In order to uncover mechanistic details of sRNA transport, it is essential to identify the protein components of the RNP complexes.
Research Strategy
It is the major aim of the exRNA program to create a vibrant, dynamic and internationally leading collaborative group with the common goal of developing a mechanistic understanding of ckRNAi between plant hosts and their interacting microbes. The central working hypothesis is that sRNAs (RNA effectors) have an evolutionarily conserved key role in the establishment and development of pathogenic and mutualistic plant-microbe interactions, and therefore have a high potential for crop plant improvement and more sustainable production.
The exRNA consortium unites experts with advanced knowledge and key publications in the field of noncoding RNAs, RNA transport, RNA-protein interaction, ckRNAi, vesicle biology, and RNA-based plant protection. Past and recent breakthrough findings in plant ckRNAi were initialized to a great part by knowledge from animal research, including the discovery of the functional role of EV-mediated RNA transfer from parasites to mammalian cells (LaMonte et al. 2012; Buck et al. 2014). Key characteristics of the exRNA consortium are the collaborative work on genetically tractable crops (barley, maize, rapeseed, tomato, tobacco, olive) along with monocotyledonous and dicotyledonous model plants Brachypodium distachyon and Arabidopsis thaliana. The biological systems were selected by the following criteria: agronomic significance, and broad representation of microbial life styles, to compare biotrophic and necrotrophic pathogens as well as beneficial fungi (Table 1).
Table 1: Biological systems and microbial life styles in the exRNA consortium
Biological system | Microbial taxon | Microbial |
---|---|---|
Piriformospora indica – Brachypodium distachyon P. indica – Arabidopsis thaliana | Fungi, Basidiomycota | beneficial |
Blumeria graminis – Hordeum vulgare Golovinomyces orontii – Arabidopsis thaliana | Fungi, Ascomycota | pathogenic |
Fusarium graminearum – Hordeum vulgare F. graminearum – Arabidopsis thaliana | Fungi, Ascomycota | pathogenic |
Botrytis cinerea – Solanum lycopersicum B. cinerea – Arabidopsis thaliana | Fungi, Ascomycota | pathogenic |
Xylella fastidiosa – Olea europaea X. fastidiosa – Arabidopsis thaliana | Bacteria, Gamaproteobacteria | pathogenic |
Ustilago maydis – Zea mays | Fungi, Basidiomycota | pathogenic |
Thecaphora thlaspeos – Arabidopsis thaliana | Fungi, Basidiomycota | pathogenic |
Verticillium longisporum – Brassica napus | Fungi, Ascomycota | pathogenic |
Mammalian cells | - | - |
The consortium will answer whether plant sRNA candidates operate on a broad spectrum of fungi (life-style dependent vs. independent), including potential trade-offs on interactions with beneficial microbes. Mutualistic symbioses and beneficial microbes are a key factor in agriculture and especially in plant production. Hence, elucidating the molecular mechanisms of mutualism in host-microbe interactions and beneficial microbes is essential for more sustainable plant production. In order to ensure further comparability of experimental data, created by the exRNA consortium, the comparative analysis of different host-microbe systems requires benchmarking of sequencing of plant and microbe transcriptomes, sRNA and proteins.
Research focus 1 – how conserved is the mechanistic role of sRNA in cell-to-cell communication during plant-microbe interactions?
A bioinformatics-based systems-level approach will comparatively analyze plant and microbial extracellular RNA from fungi, an oomycete and bacteria to determine their targets (genes and pathways) in the interacting organisms. This approach will require generation of a huge data set from concomitant sequencing of RNA biotypes (where not already available) and application of sophisticated bioinformatics analysis. The aim is to identify conserved elements of ckRNAi, potentially including species-, kingdom-, and lifestyle-specific factors. The intriguing question is whether there is a unifying model of RNA communication.
Specific questions: i. Is the current model applicable to all types of interactions, including pathogens and beneficial fungi, eukaryotic and prokaryotic microbes? ii. Are there evolutionarily conserved features present in a broad range of host-microbe interactions? iii. Does RNA communication function in the absence of a operative RNAi machinery? iv. Following a translational strategy, we will also address the question in greenhouse and field experiments whether novel sRNAs/dsRNAs and targets can be exploited in crop protection.
Research focus 2 – how does RNA move between interacting organisms?
The exRNA consortium will further test the hypothesis that EVs are key for the extracellular path(s) of bidirectional RNA exchange. Interestingly, we observed recently that even distinct mRNAs are present in EVs from infectious hyphae of Ustilago maydis, indicating that besides sRNAs also mRNAs can be exchanged between pathogen and host (unpublished data from the Feldbrügge lab). Furthermore, the consortium will assess the possibility that RBPs are critical components of the RNA loading into EVs and, thus, RNA transfer.
Specific questions: i. Are there alternative routes for RNA transfer between interacting organisms and is there an EV-independent pathway? ii. What molecular components – especially RBPs - are required for RNA loading into EVs and RNA transfer? iii. Have plants developed RNA capture mechanisms to block transfer of microbial sRNAs into plant cells? We anticipate that the knowledge gained in this research focus can be used to improve direct RNA applications. iv. Can we use novel formulations, biochemically modified RNAs, RBPs and/or vesicle-based compositions for sRNA/dsRNA delivery to plants?
Collaboration
The exRNA consortium collaborates with the German Network for Bioinformatics Infrastructure (de.NBI) on data management, software development for RNA data analysis and implementation of scalable workflows in cloud computing environments; in addition the consortium keeps a close communication with the German Society of Extracellular Vesicles by engaging the consortium in conferences and workshops. Finally, the consortium is working closely with the EU-funded ERA-NET consortium BioProtect, which develops key strategies on RNA formulations to bring RNA technologies to market as soon as possible.
Acknowledgement:
This consortium is funded by Deutsche Forschungsgemeinschaft in the programme Research Units (RU5116) grants KO 1208/30-1 to K.H.K., PA 861/22-1 to R.P., WE 5707/2-1 to A.W., RO 3550/16-1 to S.R., KE 856/8-1 to J.K.; FE 448/15-1 to M.F., BI 316/20-1 to A.B. and C.P., and GO 2037/8-1 to A.G, GO 2064/4-1 to V.G.