NTA goes co-localization: Characterization of double-labelled extracellular vesicles
Characterization of bio-nanoparticles (BNPs) such as extracellular vesicles (EVs), exosomes or virions has usually been a demanding task requiring expertise, significant time and sample amount. While nanoparticle tracking analysis (NTA) is a comparatively fast technique, it has been predominantly used for measurement of particle size distribution and concentration determination in unspecific scatter mode. With the possibility of fluorescence detection, NTA reached a milestone where fluorescently labelled particles can be detected offering enhanced resolution and bio-specific results compared to scatter-based NTA. This communication describes the most current progress of NTA: quantification of colocalization ratios of two complementary fluorescently labelled compartments present on BNPs. Technical principles of the advanced setup of a PMX-230 TWIN ZetaView® instrument with colocalization software feature are presented and experimental guidelines such as staining procedures and software settings for the characterization of fluorescently double-labelled BNPs are discussed. For the first time we present quantitative colocalization results of selected EVs (cell line and donor) and phages realized with NTA.
Keywords: Nanoparticle Tracking Analysis, Fluorescence, Labelling, Immunolabelling, Tetraspanin, Membrane dye, Colocalization, Biomarker, Exosomes, Phenotyping
Introduction
Techniques incorporating fluorescence as detection principle can be regarded as the analytical backbone in almost every bioanalytical laboratory. For the characterization of exosomes, micro vesicles (MV) and virions, resumed as bio-nanoparticles (BNPs), fluorescent staining techniques allow fast and specific staining of membranes, surface proteins or nucleic acids by applying lipid dyes, antibodies, and intercalating RNA/DNA dyes [1, 2]. The presence or absence of characteristic targets is associated with the biological function of such BNPs, therefore labelling procedures and bioanalytical techniques following labelling need to be well-matched to achieve optimum results.
In general, the term colocalization refers to the simultaneous detection of two or more specific targets in the same space. Each target is labelled with a specific fluorescent probe, having individual fluorescent excitation wavelengths and emission characteristics [3]. Quantitative colocalization measurements on BNPs are challenged by their size and the low number of available fluorochromes. Assuming an EV of 100 nm and an antibody with 3 nm in size, the number of antibodies conjugated to an EV is ~4000 for a monolayer. Presumably an average of 2.5 fluorochromes per antibody results in ~10000 fluorochromes attached to an EV. In real samples such numbers are considerably lower due to epitope density, steric hinderance, inactivation and multi-labelled EVs. To resolve such challenging characteristics with fluorescence microscopy (FM) significant enhancement of resolution and limit of detection is necessary. Stochastic optical reconstruction microscopy (STORM) provides approx. 10-fold higher resolution to conventional fluorescence microscopy and even allows resolution of the distribution of tetraspanins on a single EV in 3D [4, 5]. While characterization of blood cells or HIV is routinely performed with flow cytometry (FCM), detection of EVs in the size range of 30 – 200 nm remains challenging. Experiments need careful consideration with respect to correct EV count, neither not to miss the EV population nor to pick up noise instead of real signal. EV analysis performed by FCM started more than a decade ago and requires both high performance instrumentation and a certain level of expertise [6–9].
Nanoparticle tracking analysis (NTA) is a technique for determination of particle size distribution and measurement of concentration of in a liquid dispersed BNPs in the size range from 40–1000 nm. Since its commercialization NTA has been quickly used for the characterization of EVs [10], however, predominantly in scatter mode. This communication is dedicated to fluorescence detection with NTA and presents the principles of the most current development: colocalization measurements with an advanced PMX-230 TWIN ZetaView® instrument. Experimental guidelines for sample staining and software settings for the characterization of double-labelled BNPs will be given and results from several types of double-labelled EVs and phages will be presented.
A brief introduction into nanoparticle tracking analysis
Analogue to an ultra-microscope setup, a laser illuminates the sample perpendicular to the observation by a sensitive optical camera chip where the scattering light of the particle is detected. From the analysis of the Brownian motion of the particles, the size of each particle is determined according to Einstein’s diffusion model. Further details about NTA can be found in [10-11]. Samples typically need dilution and are directly injected through a Luer® port. Sizing of more than 2000 single particles is performed in less than a minute, with a throughput of up to 30 samples per hour. While particles are analyzed directly in dilution, no immobilization step is needed making NTA a fast and flexible platform for research.
Detection of fluorescence and determination of colocalization ratios with NTA
NTA reached a milestone when sensitive cameras working under ambient condition became readily available [12]. Switching from scatter to fluorescence mode, a long-pass filter is used to block the excitation wavelength and fluorescently labelled particles are detected allowing sizing and counting. Analogue to FCM, it is crucial to carefully match excitation properties of a photostable fluorophore to the excitation wavelength of the laser and the emission properties to the transmission curve of the long-pass filter. With the integration of several lasers, NTA offers scatter and up to four lasers to perform phenotyping of EVs and several filters [9, 13–15].
NTA channels and colocalization combinations are summarized in table 1.
Table 1: NTA channels and colocalization experiments.
| SCATTER CHANNEL | FLUORESCENCE CHANNEL 1 | FLUORESCENCE CHANNEL 2 |
---|---|---|---|
NTA CHANNEL | 488S | 488F500 | 640F660 |
EXCITATION | 488 nm | 488 nm | 640 nm |
FILTER | - | 500 nm | 660 nm |
SINGLE CHANNEL MEASUREMENT | scatter, total particle count | concentration & size green-fluorescent particles | concentration & size red-fluorescent particles |
COLOCALIZATION MEASUREMENT |
| Colocalization 640F660 - 488F500 | |
Staining efficiency 488F500 - 488S |
| ||
Staining efficiency 640F600 - 488S | - | Staining efficiency 640F660 - 488S |
The sequence of an automated colocalization experiment is illustrated in figure 2: short image sequences are recorded from 640F660 (left) and 488F500 channel (center) and an overlay is produced (right). The nomenclature for fluorescence channels follows (excitation)F(emission), for scatter the 488 nm laser was used (488S). A colocalization event occurs when a particle containing red and green fluorescence tags evokes detections in both channels within a certain proximity stretch (marked as orange circles). The proximity stretch is defined by the so-called search radius around the detections and scales with the Brownian motion of the particle, hence smaller particles will diffuse longer distances requiring larger proximity stretches and vice versa. To achieve colocalization ratios, the number of colocalization events is divided by the detections of the channels 640F660 and 488F500 respectively. The key prerequisites for quantification of such ratios are:
- illumination of identical measuring volume by both lasers
- short switching times between the channels to minimize the loss of linking partners by diffusion and
- setting of search radius to minimize false positive events.
Material and methods
Nanoparticle tracking analysis
Measurements were performed by a PMX-230 TWIN ZetaView® model equipped with two lasers 488 nm and 640 nm with corresponding long-pass filters with 500 nm and 660 nm cut-off wavelengths. The instrument was controlled with Particle Metrix Software Suite. In comparison to the standard ZetaView® Software, PMSS features expanded range of measurement parameters, sequences of user defined measurements and colocalization feature.
Table 2 summarizes the key measurement parameters.
Table 2: Key measurement parameters. All measurements were carried out with temperature control at 25 °C.
| DNA beads | F-SiO2 | EVs and Phages | ||||||
---|---|---|---|---|---|---|---|---|---|
NTA channel | 488S | 488F500 | 640F660 | 488S | 488F500 | 640F660 | 488S | 488F500 | 640F660 |
Sensitivity | 60 | 94 | 90 | 75 | 92 | 99 | 80 | 92–99 | 92–99 |
Shutter | 200 | 100 | 100 | 100 | 100 | 80 | 100 | 100–200 | 100–200 |
Noise reduction | - | 3 | 3 | - | 3 | 3 | - | 3–10 | 3–10 |
Video length | 100 | 15 | 15 | 100 | 6 | 6 | 60 | 6–15 | 6–15 |
Measurements were carried out on three positions to accumulate > 300 traces per run. Noise reduction was applied to average intensity fluctuations of pixels over 3 to 10 subsequent images and reduced false positive counts in the presence of free dye. To minimize the effect of photobleaching, the video length was set <15 frames and acquisition was carried out with low bleach option.
Particle standards
Instrument performance of size, concentration and fluorescence detection was verified with polystyrene particles 100 nm (A3100), yellow/green-fluorescent polystyrene particles (F8803), deep-red fluorescent polystyrene particles (F8807), all from Thermo Fisher Scientific, MA USA. Single (DY488 or DY643) and double-labelled silica (DY488 and DY640) particles were stained with dyes from Dyomics and custom made (Micromod GmbH, Germany), further referred to as F-SiO2 particles. If not stated otherwise, standards were diluted with practically particle free ultra-pure water (Carl Roth, T172).
Fluorescent labelling of streptavidin coated polystyrene particles
The fluorescent labelling was performed using 100 nm sized streptavidin coated polystyrene microspheres (CP01000, Polysciences Europe GmbH, Germany). The staining was realized by mixing the microspheres with fluorescently labelled and biotinylated DNA. All HPLC purified oligonucleotides were purchased from Integrated DNA Technologies, Inc., USA. In detail, 5 µl of the microspheres (0.1 % w/v) were mixed with 10 µl of 10 µM biotinylated and fluorescent labelled DNA and incubated for 1 hour at room temperature. By using DNA strands with different fluorophores, three different samples were prepared, further referred to as F-DNA-PS: Single stained particles (Alexa FluorTM 488 or Alexa FluorTM 647) and double stained particles (Alexa FluorTM 488 and Alexa FluorTM 647). 5 µl of each sample was diluted in 80 µl water and stored at 4 °C. Before injecting the particles for analysis in the NTA analyzer, each sample was diluted 1:200 with water to adjust the concentration.
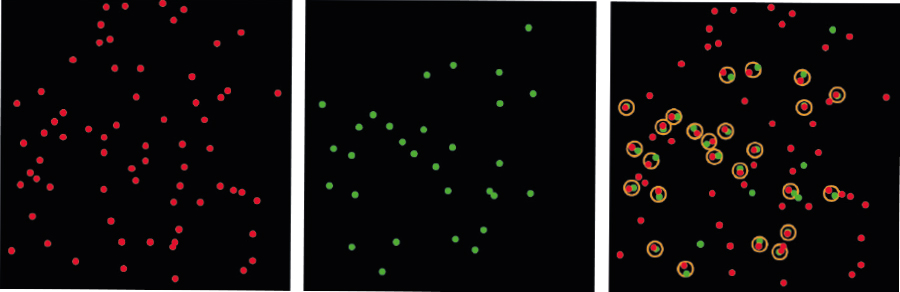
Figure 2: Sequence of colocalization experiment with NTA on double-stained vesicles: (left) Acquisition in fluorescence channel 640F660 for detection of red labelled vesicles, (center) channel 488F500 for green labelled vesicles, (right) overlay. Colocalization events are indicated with an orange circle for illustration.
Labelling of EVs and bacteriophages
If not noted otherwise, concentration of BNPs was determined prior staining and samples were diluted in PBS (GibcoTM, pH 7.4). Monoclonal anti-human antibodies and dyes (Thermo Fisher Scientific, MA USA, BD Biosciences, NJ USA and Biotium, CA USA) required predilution before conjugation with EV samples as described. Incubations were performed at room temperature (RT) in the dark.
Bacteriophage Phi6 was obtained from the Leibniz Institute (CsCl-purified bacteriophage Φ6, DSMZ, German Collection of Microorganisms and Cell Cultures GmbH, Braunschweig, Germany). For dsRNA labelling, 1 µl of SYBRTMGold nucleic acid stain (predilution 1:50) was mixed with 1 µl of the bacteriophage (4 x 1010 PFU) and incubated for 1 hour. For the second staining, 1 µl of a 1:100 dilution of CellBrite® Fix 640 was added to the mixture of step 1 and incubated for 15 min. The staining mixture was subsequently filled to 2000 μl with PBS buffer before NTA measurement.
HUVEC EVs, kindly prepared by the working group of Wolfgang Holnthoner (Ludwig Boltzmann Institute, Section Traumatology, Vienna, Austria), were used as provided [9]. 5 µl of EVs were gently homogenized after addition of 1 µl of each CD31 (predilution 1:20), CD81 (1:8) and Cell MaskTM deep red (CMDR, 1:2000). Both antibodies are directly labelled with PE-CF594. After filling to 10 µl with PBS the sample was incubated for 45 min and required a 250-fold dilution before analysis.
THP-1:CD63eGFP EVs were kindly provided by the working group of Bernd Giebel (University Hospital Essen, Institute for Transfusion Medicine) and are described in detail [8, 16]. 5 µl of the EVs were mixed with 1 µl CD63-APC (predilution 1:2, clone MEM61, Exbio, Poland) and incubated for 2 hours. The sample was filled to 1000 μl with PBS buffer and subjected to NTA measurement.
Lyophilized HCT116 EVs (HBM-HCT-100, HansaBioMed Life Sciences, Estonia) were reconstituted in water according to the manufacturer's instructions resulting in a concentration approx. 5x1011 cm-3. All antibodies required a predilution of 1:20, all incubation steps were carried out for 15 min. The EVs were stained in a three-step conjugation as follows: Step 1.) to 6 µL of reconstituted EVs unlabelled antibodies CD63 (2.5 µl) and CD81 (1.5 µl) were added respectively to achieve an incubation volume of 10 µl. After incubation, the sample was brought to a volume of 150 µl and ultra-filtrated (Nanosep® MWCO 100 kDa, Pall GmbH, Dreieich-Biotech). Centrifugation was carried out at 500 x g for 10 min (Hermle Z216M, rotor 220.87). The EVs deposited on the membrane were resuspended with PBS and transferred in a new reaction vial. Step 2.) After adding 2.5 µl of PE labelled anti mouse-IgG antibody, the sample was incubated. The unbound antibody was removed by SEC (Exo-spinTM, Cell Guidance Systems, United Kingdom) according to the instructions of the manufacturer. Step 3.) 100 µl of the washed EVs were incubated with 1 µl of 1:2000 prediluted CMDR. Incubation was stopped after 10x dilution.
Results
To ensure that both lasers (488 nm and 640 nm) are illuminating the identical volume, a series of measurements was carried out. Here, the position of laser 1 remained fixed while laser 2 was laterally shifted and colocalization ratios were determined for each increment. Figure 3 (left) illustrates a colocalization ratio > 90 % (top), representing an ideally aligned instrument.
The colours of the dots represent the particles detected with 488 nm (green) and 640 nm (red) laser respectively. Blue crosses visualize colocalization events. In contrast, misaligned lasers result in ratios < 30% (bottom). The ratios of the full course of a laser focus gives confidence to work in the optimum.
Quantification of colocalization ratios
The performance was verified by several sets of artificial mixtures, containing single and double stained particles. Figure 4 (left) shows a graphical visualization of colocalization events (blue crosses) of intrinsically stained F-SiO2 particles with descending ratio of double stained particles from top to bottom. Experiments were repeated with F-DNA-PS and F-PS particles (Figure 4, right).
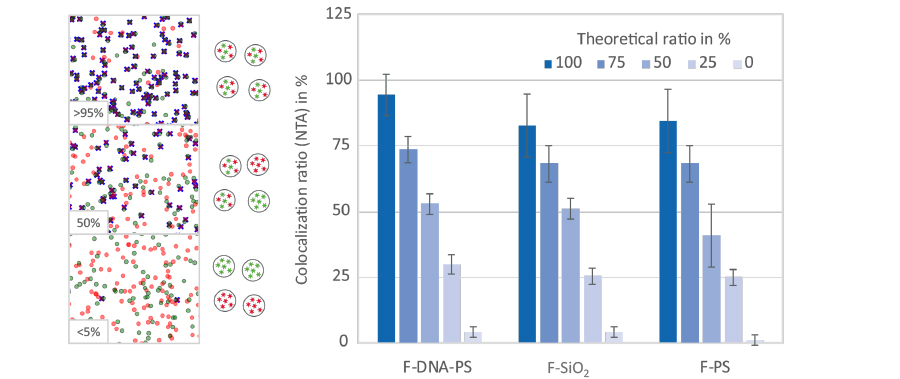
Figure 4: Screenshots from scatter plots of mixtures of F-SiO2 with increasing colocalization ratio (left). The colocalization ratios quantified with NTA are in good agreement with the theoretical mixtures for all three scenarios (right).
The colocalization ratios quantified with NTA were in very good agreement in comparison to the theoretical mixtures within the experimental error. This shows clearly that colocalization ratios can be quantified with an NTA setup as well as the standards used are fit for purpose.
While the ratios > 50 % agree well for F-DNA-PS particles, the ratios of F-SiO2 and F-PS are somewhat lower than expected. For F-SiO2, the signal obtained in the 640F660 channel was noticeable weaker compared to 488F500. The difference in counts in the red channel could be attributed to an uneven distribution of fluorophores per particle and more likely a lower photostability of DY643.
Analysis of double-stained bio-nanoparticles
Table 3 summarizes the targets and the corresponding NTA channels of all four sets of double labelled BNPs with EVs and phages.
Table 3: Summary of double lablelled biological vesicles.
NTA Channel | Phi 6 | THP-1 EVs | HUVEC | HCT116-EVs |
---|---|---|---|---|
488F500 | SYBRTMGold (nucleic acid dye) | CD63-eGFP | CD31 + CD81 primary AB directly labelled PECF594 Sum parameter | CD81 + CD63 Secondary AB Anti-mouse labelled with PE Sum parameter |
640F660 | CellBrite® 640 (Plasma membrane dye) | CD63-APC | Plasma membrane dye | Plasma membrane dye |
Figure 5 shows the result of a full fluorescence analysis of double stained HUVEC EVs.
Detection in unspecific scatter mode resulted in 140 nm peak size. Staining efficiencies (Figure 6) calculate as ratio of concentration in fluorescence channel over total particle concentration, assuming labelling of EVs with the membrane dye.
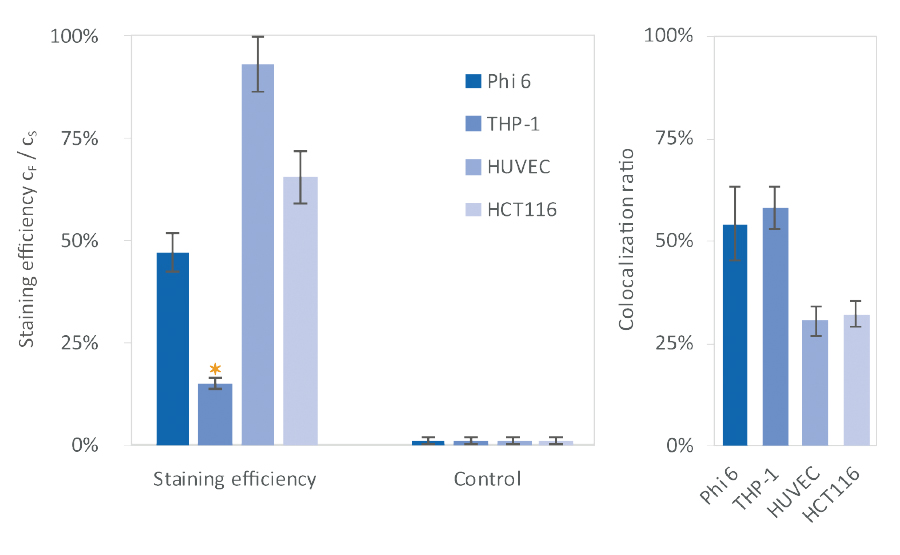
Figure 6: Membrane dyes as unspecific vesicle marker yielded staining efficiencies >50%. The lower sample purity of the THP-1:CD63eGFP EVs (marked with an asterisk) resulted in lower staining efficiency. Colocalization ratios were presented for the first time for cell line EVs and EVs harvested from patient samples.
With the 640 nm laser, a high staining efficiency of 93 % ± 3 % (n = 3, m = 3, 1s) was achieved (with number of technical replicates n, number of measurements per replicate m and standard deviation s). All samples, summarized in table 3, showed the same trend in concentration and peak sizes: concentration was always highest in scatter followed by membrane staining and antibody staining. While samples showed the same trend of the peak from largest in scatter to smallest peak size, the differences of peak sizes remained within +/- 30 nm.
The THP-1:CD63eGFP EVs showed a staining efficiency of 15 % ± 8 % but showed the highest colocalization ratio of 58 % ± 5 %. Other techniques (oral communication, results not shown) confirm the trend. In order to avoid common pittfals of membrane dyes [17,18], proper application of dyes and design of controls ensure that the dye itself is not contaminating the sample with fluorescent vesicles. We postulate that membrane dyes serve as an estimate for sample purity, together with the prior knowledge about the sample. Especially in the beginning of setting up a SOP for fluorescence characterization of EVs it is vital. The HCT116 EVs were labelled with a three-stage labelling protocol. When labelling with secondary antibody, the removal of the unbound primary antibody is of great importance. Even remaining traces can form antibody agglomerates, which form a subpopulation around 30–60 nm interfering with the major peak (data not shown). Considering the controls, the removal of unbound primary antibody was successful and a colocalization ratio of 28 % was achieved.
Summary and concluding remarks
In summary, essentials of fluorescence detection with NTA were presented laying the foundation of colocalization measurements. Colocalization ratios determined by NTA were in excellent agreement with those of artificial mixtures, confirming fitness of standards as well as instrumentation. Several staining procedures for double-labelling of EVs derived from HUVEC, THP-1 and HCT116 cells and phages were presented involving membrane and nucleic acid dyes as well as fluorescently labelled antibodies. The automatic processing of measurements via scripts ensures fast acquisition under controlled conditions, minimizing sample amount and increasing unsupervised instrument time maintaining a working range down to 40–50 nm for BNPs. For the first time, quantitative colocalization rates of double labelled EVs and phages are reported, making NTA a fascinating fast tool for phenotyping of BNPs.
Acknowledgements
The authors thank Bernd Giebel for THP-1:CD63eGFP EVs and Wolfgang Holnthoner for providing HUVEC EVs for the development of the double labelling protocols and fruitful discussions with the members of the workinggroups. Additionally, we thank Sven Kreutel and Ray Eby for the careful proof-reading of the manuscript.