Crossroads of the endosomal machinery:
Multivesicular bodies, small extracellular vesicles and autophagy
The primary role of endosomal system is endocytic trafficking – to sort out internalized macromolecules and proteins to their destined cellular localizations. Incorporation of sorted cargos into multivesicular bodies (MVBs) confers specificities and determines their fates. This central point of the endosomal trafficking separates MVBs in two directions. The MVB populations fuse either with lysosomes to initiate autophagy or with plasma membrane to release small extracellular vesicles...
Keywords: Exosomes; degradative MVB; secretory MVB; physiological stress; endocytic machinery; lysosome
Introduction
Endosomal system is a complex network of membrane compartments, including early endosomes, recycling endosomes, late endosomes/multivesicular bodies (MVBs) and lysosomes. These sub-cellular compartments are interconnected with each other and the plasma membrane by highly regulated trafficking pathways. The endosomal network provides a sorting, processing, recycling and degradation platform with cytoplasmic machineries for a diverse array of internalized cargos on the luminal side, such as proteins and lipids. Being the receiving point of incoming cargos and the first sorting hub, the early endosome selects and delivers cargo to recycling endosomes, trans-Golgi network or MVBs [1]. In this review, MVB sorting and trafficking is discussed with special emphasis on small extracellular vesicle (sEV) secretion and autophagy.
MVBs: the crossroad
Evolving from progressive maturation of early endosomes, MVBs are typified by increased number of intra-luminal vesicles (ILVs). Discrete sorting mechanisms (endosomal sorting complex required for transport (ESCRT)-dependent/-independent) participate in incorporation of specific cargos into ILVs, thus leading to distinct sub-populations of MVBs. The ESCRT machinery comprises 20 proteins which are assembled into four different complexes (ESCRT-0, I, II and III) along with their associated proteins. Sequential recruitment of ESCRT complexes on MVBs leads to inward budding of its limiting membrane and subsequent abscission to form ILVs within MVB. During this process, ESCRTs can incorporate ubiquitinated or sumoylated cargos into ILVs [2, 3]. In contrast, ESCRT-independent pathway operates in ceramide-induced manner [4]. Additionally, an accessory ESCRT protein, ALIX plays an important role in ILV biogenesis within MVBs [5–7]. These MVB sub-populations have defined fates along the endocytic pathway. Thus, MVB biogenesis is a crucial point, where the endosomal system functionally diverges into two routes, the degradative or secretory pathways (Figure 1).
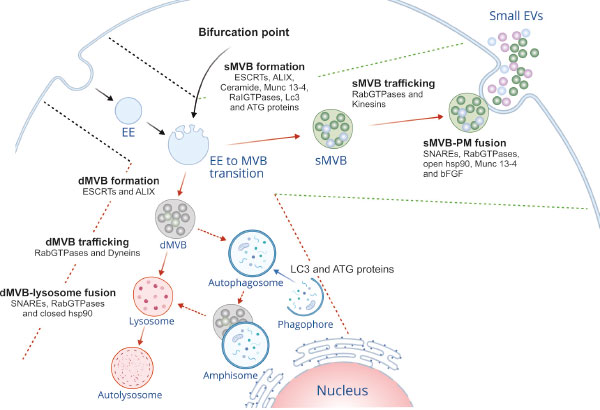
The functional bifurcation is attributed to the type of cargo harbored by MVBs. For example: MVBs containing ubiquitinated epidermal growth factor receptor are targeted to lysosomes for degradation [8]. In contrast, cholesterol-laden MVBs tend to fuse readily with plasma membrane (PM) to liberate sEVs [9]. Interestingly, MVBs containing ubiquitinated major histocompatibility complex (MHC) class II undergo lysosomal degradation in immature dendritic cells. In contrast, non-ubiquitinated MHC-II – and CD9 – bearing MVBs are targeted for PM fusion to secrete sEVs in T-cell activated dendritic cells [10].
More and more the molecular basis of these alternative fates of MVBs is emerging. It is now apparent that various regulatory molecules as well as molecular switches govern the segregation of differential cargo-laden MVBs. Late endosomal small GTPases, such as Rab7 and Arl8b exhibit opposite functions depending on their effectors and motor proteins. Rab7 via its effector RILP recruits the dynein motor protein (minus end-directed transport), which leads to perinuclear accumulation of MVBs. Later, it leads to lysosomal degradation of cargo by recruitment of HOmotypic fusion and Protein Sorting (HOPS) complex. By contrast, Arl8b entails accumulation of MVBs at cell periphery (plus end-directed transport) with the help of kinesins recruited by effector SKIP. Thus, molecular switching of Rab7-to-Arl8b on MVB decides the ultimate fate of MVBs [11]. Cell peripheral accumulation of MVBs by ordered Rab7-to-Arl8b handover might have a role in sEV secretion, which requires further studies. Nevertheless, Ar18b has also been shown to promote MVB-lysosome fusion depending upon its effectors. Apart from SKIP, Ar18b has another effector PLEKHM1. Along with its effector PLEKHM1, Ar18b facilitates clustering and fusion of MVBs with lysosomes [12]. Another bifurcating factor of MVB trafficking is Tetraspanin-6 (TSPN6). Interestingly, TSPN6 directly interacts with syntenin, a sEV-sorting determinant [13]. However, here the level of protein is the fate-determining. When cellular levels of TSPN6 are low, syntenin and syndecan (SDC) 4 cargos are en-routed to secretory MVBs. In contrast, high levels of TSPN6 direct the same cargos to lysosomal degradation pathway [14].
Secretory MVBs: the dump
Secretory MVBs are the subpopulation of MVBs en-route to PM fusion to release their ILV pools into the extracellular space as sEVs or exosomes. Correctly regarded as eliminated cellular contents or wastes, these vesicles serve in intercellular communication to influence development and disease. ESCRT machineries actively participate in protein sorting and formation of ILV within secretory MVBs [15]. It has been shown that the ESCRT complex utilizes sumoylation (SUMO) as a sorting signal along the endosomal pathway. SUMO in conjunction with phosphoinositols operates to separate the α-Synuclein and subsequently liberate α-Synuclein enriched sEVs [3]. The ESCRT-I component Tsg101 binds a tetrapeptide motif (P(S/T)AP) of galectin-3 to sequester it into ILVs of secretory MVBs [16]. Similarly, the ESCRT-III protein CHMP1A is required for ILV biogenesis within MVB and secretion of sonic hedgehog-positive sEV subtype [17]. In contrast, the ESCRT-independent pathway involves the hydrolysis of sphingomyelin into ceramide at the MVB membrane, which is mediated by the action of neutral sphingomyelinases (nSMases), resulting in the budding of ILVs internally into the secretory MVB [4].
As mentioned above, ALIX provides an additional pathway of ILV formation. In reticulocytes, ALIX interacts with transferrin receptor to sort it to MVB, which are eventually released as sEVs [15]. A recent report has shown that besides interacting with lysobisphosphatidic acid (LBPA) that was exclusively found in late endosome, ALIX mediates recruitment of ESCRT-III to late endosomes. This facilitates endosomal sorting of tetraspanins into MVBs and their secretion via sEVs [6]. Additionally, specific scaffold proteins such as syndecans (SDCs) heparan sulfate proteoglycans are implicated in formation and cargo loading of secretory MVBs. SDCs via their cytosolic adaptor syntenin form a complex with ALIX that binds several ESCRT proteins [18]. SDC-syntenin-ALIX axis adapts SDC cargo to the ESCRT machinery at the MVBs. Consequently, segregation of SDC cargo into ILVs of secretory MVBs is achieved. Heparan sulphate seems to be actively involved in the process. SDC cargo may possibly interact with SDC via heparin sulphate leading to formation of SDC assembly, which in turn recruits syntenin-1 and ALIX, thus fostering ILV budding [5]. Moreover, heparanase has been shown to cleave and activate the heparan sulfate chains of the SDCs. This entails enhanced SDC clustering and promotes ILV budding by modulating SDC-syntenin-ALIX pathway [19]. Functioning upstream of ADP-ribosylation factor 6 (ARF6) GTPase and its effector phospholipase D2, the cytoplasmic tyrosine kinase SRC has been shown to affect not only the syndecan cargo endocytosis but also the syntenin–syndecan ILV formation by directly phosphorylating the conserved juxta-membrane DEGSY motif of the syndecan cytosolic domain and syntenin tyrosine 46 [13]. This suggests the existence of additional layers of regulatory mechanisms on functional bifurcation of the endosomal system.
The next step of secretory MVB biogenesis is trafficking towards PM. RabGTPases such as, Rab27a and Rab27b and as mentioned, kinesin motor proteins in association with Arl8b and its effectors SKIP/PLEKHM2 mediate transport of MVBs to the cell periphery [11, 20]. Subsequently, the MVBs tether and dock to the PM, releasing their ILVs as sEVs. Rab27a, Rab27b and Rab35 play pivotal roles in MVB-PM fusion [20, 21]. Interestingly, Rab27a has recently been shown to be regulated by KIBRA, which stabilizes Rab27a by preventing its ubiquitin-proteasomal degradation. This adds extra layer of regulatory mechanism in the docking event [22]. Likewise, Ral GTPases are other important regulators of MVB formation and sEV release both in Caenorhabditis elegans and mammals. In C. elegans, RAL-1 regulates both budding of MVBs and their fusion with PM [23]. The fusion event is mediated by Syntaxin-5 (Stx5), a soluble NSF-attachment protein receptor (SNARE) family protein. SNAREs are fusion engines that control the fusion between different membranes, and as such, MVBs and the PM. The v-SNARE VAMP7 and ATPase NSF, a protein required for SNAREs disassembly are essential for MVB-PM fusion in human K562 cells [24], while the r-SNARE Ykt6 does the same in both mammalian and Drosophila cells [25].
In addition, Ykt6 depletion has been shown to impair the secretion of Wnt bearing sEV subtypes in the above settings [26], further suggesting its active involvement in secretory MVB route. Synaptosomal-associated protein 23 (SNAP-23) is a t-SNARE protein that specifically mediates the fusion of secretory MVB with the PM upon histamine-mediated activation of GPCR signaling, consequently releasing CD63-bearing sEVs [27]. Munc13-4 is a Ca2+-dependent SNAP receptor- and Rab-binding protein. Munc13-4 causes formation of secretory MVBs by controlling membrane type 1 matrix metalloproteinase-P sorting via the Rab11 pathway, maturation of CD63-bearing MVBs and Ca2+-dependent membrane fusion, consequently triggering sEV release in cancer cells [28]. The chaperon heat shock protein Hsp90 has been shown to specifically mediate the fusion of MVBs to PM. The open conformation of Hsp90 deforms membranes and favours fusion leading to sEV release, while the closed Hsp90 blocks this event [29]. Growth factors greatly impact the secretory MVB-PM fusion, in cultured hippocampal neurons. Basic fibroblast growth factor (bFGF) was demonstrated to promote the rate of stimulus-evoked MVB-PM fusion leading to increased release of VAMP3 and cellubrevin bearing sEVs [30].
Degradative MVBs:the recycling plant
Subsets of MVBs – degradative MVBs – are destined to fuse with lysosomes. Upon heterotypic fusion, MVBs deliver their ILVs to the lysosome for degradation through autophagy. The MVB-related autophagy happens in two ways – macroautophagy and microautophagy. Macroautophagy degrades damaged organelles and MVBs by first incorporating them in autophagosomes to form amphisomes, which in turn fuse with lysosomes. In microautophagy, autophagic cargos are directly internalized into the ILVs of MVBs. In both kinds of autophagy, ESCRT machinery plays a crucial role in cargo loading to the degradative MVBs [31], including ubiquitinated integral membrane proteins and cytosolic proteins harboring a targeting motif (KFERQ) for chaperone-mediated autophagy [2, 32]. However, not all cargos use ubiquitin or the canonical ESCRT machinery for MVB sorting. The adaptor protein complex-3 sorts signaling receptor PAR1 to degradative MVB in a manner independent of ubiquitination and ESCRTs, but dependent on ALIX pathway [7].
Once the degradative MVBs are formed, the next step is their transport and fusion with lysosomes. As mentioned, Rab7 and Arl8b are powerful players in this context sharing PLEKHM1 as effector. Rab7 mediates membrane recruitment of PLEKHM1, which in turn interacts with Arl8b. Subsequently, Arl8b mediates recruitment of HOPS complex by PLEKHM1 at vesicle contact site of MVBs/lysosomes, promoting their tethering and ultimately degradation of MVB contents [12]. Since lysosomal degradation entails both endocytic and autophagic pathways, it is plausible that they share the molecular players. Rab2 and Rab7 are two of such regulatory molecules which coordinately boost the fusion of autophagosomes and MVBs with lysosomes in HOPS-dependent manner in both mammals and Drosophila [33]. Recently, YAP/TAZ, transcriptional co-activators have been shown to regulate the fusion of autophagosomes with lysosomes [34]. YAP is partially associated with the recycling endosomal membranes in low-density cells and activated by endosomal phosphatidylserine [35]. Given its role in lysosomal fusion and association with endosomal system, it is conceivable that YAP/TAZ might also regulate the MVB-lysosomal fusion. The fate of degradative MVBs appears to be decided by multilayered regulatory machineries. Rab7A controls trafficking of ubiquitinated EGFR to degradative MVBs. Adding another layer of complexity, CD44s (isoform of CD44 lacking all variable exons) has been recognized as an upstream regulator of Rab7a, eventually modulating EGFR degradation [36].
Physiological stress such as nutrient deprivation and inhibitor treatment can trigger degradative MVB biogenesis as well as their fusion with lysosomes. Upon amino acid deprivation stress, ESCRT-III delivers autophagy receptors to degradative MVBs leading to their destruction [37]. Similarly, in yeast ESCRTs have been shown to sort aberrant proteasomes into microautophagic vesicles (ILV-like structures) of vacuole upon glucose starvation, in dependence of AMP-activated protein kinase (AMPK) [38].
Autophagy and sEV secretion-A relief route?
Autophagy is intimately associated with secretion of sEVs. Besides performing conventional degradative functions, autophagy entails extracellular secretion of cytosolic protein or sequestered material from the cells [39]. Correctly regarded as secretory autophagy, it engages both autophagy and endocytic machineries to selectively transport and release cargos to the cell exterior. Evidence suggests that IFN-γ-stimulated autophagy regulates exosomal secretion of Annexin A2 (Anxa2) in lung epithelial cells. The fusion between Anxa2-positive autophagosomes and MVBs has been shown to be mediated by Rab11, while that of Anxa2-positive amphisomes and PM via both Rab8A and Rab27a. Indeed, both Atg5 and Rab11 are essential for IFN-γ-triggered Anxa2 secretion [40]. It has been shown that inhibition of phosphoinositide 3-kinase (PI3K) complex, which plays a central role in autophagy, reduces exosome secretion in chronic myeloid leukemia [41], further arguing the link between both the pathways. Moreover, ATG proteins, central regulators of autophagy, appear to be involved in controlling sEV release. ATG12-ATG3 complex has been shown to bind ALIX to control sEV secretion [42]. Similarly, ATG5 and another autophagy related protein LC3 coordinately regulate MVB acidification and sEV production via control of ATP6V1E1 interactions with the V1V0-ATPase complex. Also, LC3 as well as several Atg proteins have been found on sEVs [43], thus strengthening the connection between autophagy and sEV production. Notably, the LC3-conjugation machinery is responsible for specific packaging and release of ribosome binding proteins and small non-coding RNAs into sEVs. The LC3-dependent EV loading and secretion (LDELS) has been shown to be mediated by nSMase2 and factor associated with nSMase2 activity (FAN) [44].
Being engaged in MVB sorting, trafficking and fusion events, certain molecular players are shared between sEV secretion and autophagy pathways, and are thus common regulators. For example, ESCRT proteins not only regulate MVB sorting, but also autophagy processes (reviewed in [31]). ESCRT-I and III has been shown to deliver soluble cytosolic proteins into the MVBs for degradation mediated via Hsc70 leading to endosomal micro-autophagy [32]. Similarly, ESCRT machinery is responsible for sequestration of mitochondria into Rab5-positive early endosomes for endosomal mediated degradation [45]. It has been shown that ESCRT machinery operates in scission of the lysosomal membrane leading to microautophagy of ER in yeast [46]. Likewise, ESCRT-III components (CHMP2A [47] and CHMP4B) have been appreciated as crucial regulators of phagophore sealing during autophagy/mitophagy [48]. Another important common regulator is SNAREs family. While the MVB-autophagosome fusion is mediated by VAMP3, a v-SNARE promoting autophagy, the MVB-PM fusion is favoured by another v-SNARE, VAMP7 [24]. Interestingly, Syntaxin (Stx)17 [49], Ykt6 [50] and SNARE priming (mediated by NSF and αSNAP) [51] have been identified to trigger the autophagosomes-lysosome fusion promoting autophagic flux. Moreover, Stx17 interacts with Stx16, which along with its cognate SNARE partners (Vti1a and Stx 6) in turn binds Atg8 via LC3-interacting regions (LIRs) [52]. Thus, it plays an important role in lysosomal biogenesis as well as autophagic maturation. In contrast, it is evident that VAMP3, Ykt6 and SNAP-23 function in the MVB-PM fusion driving sEV secretion [25, 27]. Similarly, Stx5 in C. elegans has been shown to direct secretory MVBs to the PM through Ral-1 small GTPase [23]. Given the importance of small GTPases in the endocytic system, the function of ARF6 in autophagy and sEV secretion is noteworthy. ARF6 not only facilitates ILV budding and syntenin bearing sEV secretion, but also regulates post-internalization degradation of EGFR via lysosomal fusion, arguing its dual role [53]. Besides MVB trafficking, specific Rab, such as, Rab5 GTPase module seems to be essential for autophagosome sealing [54]. It recruits ESCRT-III subunit Snf7 to Atg17-decorated autophagosomes, where the ESCRT promotes autophagosome closure leading to autophagy [55]. As mentioned, the Rab7-Ar18b switch acts as a connecting link between degradative and secretory MVB trafficking [11]. Indeed, TSPN6 has been demonstrated to function as a bridge guiding MVBs towards either degradative or secretory routes [14]. Ceramide, a cone-shaped lipid has been shown to regulate ILV budding in secretory MVBs, while LBPA, another cone-shaped lipid appears to be involved with ILV formation in degradative MVB [4]. In summary, the same or similar player(s) in the endocytic network could operate in a context-dependent manner leading to differential functional outcomes.
Conclusion
The function of endosomal organelles is highly orchestrated by numerous molecular players. Progressive transformations of early endosomes give rise to MVBs enriched in ILVs. At this critical step, distinct players decide in a context-dependent manner the kind of MVBs to be formed; either degradative or secretory. Based on the cargo loaded into the ILVs and the machinery involved at the MVBs, the endosomal network is bifurcated into two functional routes - autophagic degradation or sEV secretion. Even though specific mediators are attributed to each route, common mediators are also known to operate, suggesting that the functional bifurcation could be more complex than it appears. Since alteration in both autophagy and sEV secretion is linked to many pathological conditions, further investigations will allow to unwind the complexity behind selective MVB biogenesis and trafficking.