Extracellular vesicles as biomarkers for diagnostic and prognostic application in cardiovascular disease
Cardiovascular disease (CVD) remains the leading cause of morbidity and mortality worldwide. Early diagnosis and the development of a prognosis is important for management or secondary prevention of the disease. In the past few decades, various biomarkers have been identified for improved risk assessment, more accurate diagnosis and prognosis, and a better understanding of the underlying pathophysiology in CVD. Extracellular vesicles (EVs) are thought to be important to cell-to-cell communication in the heart, and EV counts, cellular origin, and EV content have been related to CVD. This review examines current evidence for the potential application of EVs as a new class of biomarkers in CVD.
Keywords: extracellular vesicles, biomarker, liquid biopsy, cardiovascular disease, myocardial infarction, heart failure, pulmonary arterial hypertension
Introduction
Biomarkers play a central role in the daily clinical routine and in biomedical research, delivering clinically relevant information about pathological characteristics of a disease. As such, biomarkers need to fulfil important criteria before being used in a clinical setting [1]. Even though advances in translational and clinical research have been made, there are still significant gaps that call for more reliable and innovative biomarkers for diagnosis, prognosis, risk stratification, and disease monitoring as well as the identification of novel therapeutic targets in CVD. Multiple classes of biomarkers exist and many more are under investigation. Most of them are analytes measurable in blood such as circulating peptides and proteins, metabolites, and circulating nucleic acids [2].
Over the last few years, progress has been made in analytical methods that allowed the identification of novel circulating molecules and subcellular units. Subcellular particles and their manifold features have revolutionized this field of research. As such, extracellular vesicles (EVs) have been described as novel “liquid biopsy” biomarkers in a variety of diseases [1].
EV research is a highly dynamic field, and EV nomenclature has been used quite randomly in the past. The European Society of Cardiology (ESC) defines EVs as particles ranging from 40 nm to several microns in size [3]. EVs are divided into various subgroups characterised by their size and biogenesis: (i) exosomes that are released by exocytosis of multivesicular bodies (50-150 nm); (ii) microvesicles, also called microparticles or ectosomes, that are shed from the plasma membrane (0.1-1 µm); and (iii) apoptotic vesicles, or apoptotic bodies, that are released by blebbing from apoptotic cells (>1 µm) [3]. The International Society for Extracellular Vesicles (ISEV) recently updated their guidelines on minimal information for studies of extracellular vesicles (MISEV) and recommends that the term “extracellular vesicles” should be used as the generic term for lipid bilayer-delimited particles released from the cell [4]. Thus, EV subtypes are defined by physical characteristics such as size (“small EVs” <100 nm, “medium EVs” <200 nm, or “large EVs” >200 nm) or density (low, middle, high) or biochemical composition (e.g. CD63+/CD81+ EVs) or the description of cellular origin (e.g. endothelial EVs).
The lack of clarity about the designation and characterisation of EVs in the past makes it difficult to validate results from previous studies and to assess their relevance in the biomarker field. The new definitions should allow more precise and reproducible research for the identification of EV-based biomarkers in the future, as clear descriptions of isolation, enrichment, and analytical techniques will be required [4]. This history of imprecision in EV characterization notwithstanding, multiple studies in the field of EVs have produced fascinating findings in the past two decades. In particular, it has become clear that EVs are essential for the maintenance of intercellular communication, serving as vehicles for horizontal gene transfer between cells [5, 6].
Extracellular vesicles in the heart and vasculature
The heart is a continuously contracting organ that supplies blood and nutrients to the entire body. Well-organized intercellular communication between different cell types of the heart is required to facilitate proper functioning such as myocardial contraction, vascular perfusion, and immune responses [7, 8]. Disturbances in the function and regulation of the communication network can lead to CVD.
EVs play an important role in cardiac physiology and in the pathological processes related to CVD. EVs are released from all major cell types in the heart and vascular system, including cardiomyocytes, endothelial cells, fibroblasts, and vascular smooth muscle cells. Immune cells such as monocytes, neutrophils and lymphocytes also release EVs, and platelet- and erythrocyte-derived EVs can be detected in the myocardium [3, 8-10].
The release and the vesicular composition of proteins and nucleic acids depends on the cell type of origin and are influenced by pathophysiological conditions [11-13]. Their origin from specific cell types can be determined based on characteristic combinations of specific surface markers, which also plays a role in determining downstream effects on the target cell [14].
EVs have been implicated in the pathogenesis of different stages of CVD [15], including myocardial infarction (MI), heart failure, and atherosclerosis [16]. They have been shown to regulate apoptosis and coagulation [17] and to function in an atheroprotective manner by actively contributing to heart repair in ischemia [18].
EVs derived from CD34+ hematopoietic stem cells contribute to hypoxia-induced angiogenesis and improved cardiac function after MI [19]. Interestingly, EVs have been suggested to mediate ischemic preconditioning and postconditioning in cardiac injury [20]. Upon acute MI the number of circulating cardiac EVs, mainly of cardiomyocyte and endothelial cell origin, is tremendously increased. EVs accumulating in the ischemic myocardium are rapidly taken up by infiltrating monocytes and regulate local inflammatory responses [10].
Biomarkers in CVD
Development and progression of CVD can be monitored by detection of secreted factors and the characterisation of the status and numbers of cells in the heart and periphery [21].
An early and accurate diagnosis of the underlying condition is required to apply the appropriate treatment strategy to manage CVD. Within the past few decades, treatment strategies have been improved and novel platforms for early detection and possible prevention of CVD have been investigated, leading to the discovery of various CVD-related biomarkers.
Current gold standard
The determination of cardiac troponin and natriuretic peptide levels in serum has become the gold standard for the diagnosis of CVD. These biomarkers have revolutionized diagnosis and management of acute coronary syndrome and heart failure [2]. Both markers reflect myocardial load and cardiac injury but lack the specificity to classify acute MI (e.g. type 1 vs. type 2). Although other biomarkers have not reached this level of reliability, sensitivity, and accuracy in CVD diagnostics, combination with additional markers such as myeloid-related protein 8/14 (calprotectin) has been shown to provide such discriminatory information [22]. Processes involved in CVD such as inflammation, oxidative stress, neurohormonal activation, renal dysfunction, and fibrosis offer additional biomarker sources that may improve classification and risk stratification [23].
Extracellular vesicles as biomarkers for prognosis and diagnostics
EVs bear a high potential as diagnostic and prognostic biomarkers because they can be detected in blood, pericardial fluid, and many other bodily fluids [3]. Proteins, lipids, and nucleic acids are incorporated into EVs and protected from degradation (Figure 1).
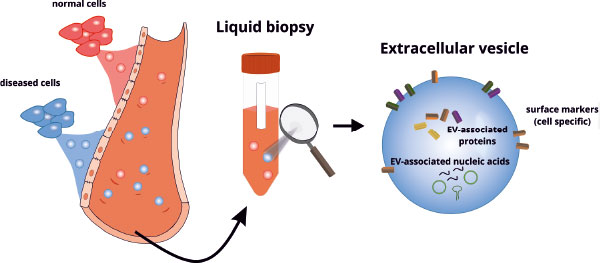
The composition is highly complex and depends on the physiological/pathophysiological status of the cells. EV populations are highly heterogeneous with regard to size, biogenesis, and cellular origin. This phenomenon is often described as a major challenge in EV-based biomarker development; however, this heterogeneity might be able to be leveraged for biomarker identification by understanding the complex composition of these EV populations. Thus, analysis of specific characteristics will be important in the identification of reliable EV-based biomarkers. Proteins incorporated into the membrane of EVs enable the determination of their cellular origin, which provides unique information [14].
Extracellular vesicle counts and cellular origin
EV numbers are associated with the presence of cardiac injury and correlate with the severity of the disease. Processes such as inflammation, vascular dysfunction, and cellular activation lead to an increase of specific EV subpopulations. Thus, EVs from various sources, mainly endothelial cells or platelets, are elevated in the peripheral blood of patients with CVD. Endothelial EVs (CD31+/CD42b-, described as microparticles) in combination with circulating miR-92a have the potential to provide diagnostic value for acute MI [42]. EVs derived from activated platelets expressing CD62P and monocytic EVs were associated with the risk of future cardiovascular events after MI [24-29].
Similar observations have been made in patients undergoing severe cardiac remodelling and vascular dysfunction that lead to heart failure. Primarily monocytic EVs (CD14+) were associated with heart failure with preserved ejection fraction, whereas endothelial EVs (CD144+) were described as being predictive of future cardiovascular events specifically in heart failure patients with reduced ejection fraction [30].
In right heart disease EV numbers may also be useful as biomarkers [31]. Pulmonary arterial hypertension is characterized by increased pulmonary arterial blood pressure and remodelling of pulmonary arterial circulation, leading to development of right ventricular failure and death [32]. A direct correlation between tricuspid annular plane systolic excursion (TAPSE), a parameter for assessing systolic right heart function, and urinary endothelial EVs was described [33]. An increase in inflammatory cell-derived EVs such as T cell EV (CD3+) was observed in various different clinical forms of pulmonary hypertension, whereas an increase in endothelial EVs (CD62e+) occurs to a different extent in different forms of pulmonary hypertension [34] and also reflects the severity of disease. Thus, CD62e+ EVs predict poor outcome in pulmonary hypertension [35, 36].
However, other comorbidities may alter the number of EVs and it is likely that EVs counts of each type are changed in multiple pathologies. As such, EV counts might indicate a more generalised injury rather than a specific pathological condition.
Extracellular vesicle content
The analysis of the EV cargo offers a fascinating opportunity to obtain information about the pathological state of the originated cells and the current state of the disease. As such, the disease-specific composition of proteins or nucleic acids associated with EVs is now often described as potential biomarker in CVD.
Circulating miRNAs such as miR-208a, miR-133a, and miR-499 have been proposed as diagnostic biomarkers in CVD [3]. However, none of them have fulfilled the requirement for clinical
application thus far. Free circulating miRNAs differ from EV-associated miRNAs in the blood. Thus, EV-associated miR-126 and miR-199a were identified as indicators of vascular injury and the occurrence of cardiovascular events in patients with stable coronary artery disease, whereas an upregulation of these free circulating miRNAs was not observed [3, 37].
EV-associated miR-183, which is involved in the regulation of protein kinase activity, is upregulated upon MI, and its level correlates with the degree of myocardial ischemic injury [38]. Furthermore, patients with acute MI showed increased serum levels of miR-192, miR-194, and miR-34a, which particular associated with EVs. These EV-associated miRNAs predict the development of heart failure and are indicative of cardiac remodelling after MI [39].
Other nucleic acids are also associated with EVs. Circular RNAs (circRNAs) are selectively packed into EVs and released into the bloodstream [40, 41]. Initial studies have revealed an association of EV-circRNAs with CVD [42]. EV-associated long non-coding RNAs such as PUNISHER, GAS5, MALAT1, and H19 were increased in patients with stable coronary arterial disease or acute coronary syndrome compared with individuals with non-coronary artery disease [43]. Future validation studies will be required to show their potential as biomarkers in CVD.
Recently, our laboratory identified a specific EV-associated P-element-induced wimpy testis (PIWI)-interacting RNA (piRNA), DQ593039, as a potential biomarker for pulmonary hypertension and right heart disease. Serum levels of EV-associated DQ593039 in patients suffering from pulmonary hypertension were significantly correlated with clinically relevant parameters [44], whereas EV-associated miRNAs that were previously described in mouse models, including miR-19b, miR-20a, miR-20b or miR-145 [45], were not different.
Furthermore, proteomic profiling showed the pathologic related enrichment of specific EV-associated proteins. As such levels of EV-associated proteins such as cystatin C, CD14, serpin F2, and serpin G1 are indicators of future vascular events [46]. The levels of these proteins (depending on the clinical scenario) showed a linear relation to increased risk of vascular events, vascular mortality, and all-cause mortality in patients with vascular diseases and heart failure. EV proteins are also associated with CVD risk factors. EV-associated cystatin C levels correlated positively with obesity, whereas CD14 levels correlated negatively, and EV-associated proteins in plasma indicated a proinflammatory and prothrombotic state in obese patients [46].
Limitations
Major limitations for the application of EV-based biomarkers include technical challenges faced in the isolation and characterisation of EVs, reproducibility, and the definition of gold-standard methodology. The typically low yields of EVs and the isolation of heterogeneous populations associated with lipid contamination represent major challenges. Furthermore, most methods are time consuming compared with determination of current diagnostic biomarkers such as high-sensitivity cardiac troponins. However, numerous laboratories are constantly working on innovative, high-resolution, and robust techniques. To promote clinical translation and adoption of these methods, technological developments will be required to advance and facilitate sensitive detection of EVs with minimal or no pre-analytical purification. In the meantime, societies such as the ISEV, German Society for Extracellular Vesicles (GSEV), and the ESC are working on the definition of standardised methods in this field in an effort to increase reliability and validity of data.
Conclusion and perspectives
Current studies have demonstrated that EVs have a great potential to evolve into biomarkers for the prediction of cardiovascular events and for the diagnosis of CVD. However, a considerable amount of research still needs to be carried out before the clinical application of EVs as biomarkers can be realised. The EV research field is still juvenile and strong societies have been founded to bring together scientists and clinicians to address the challenges in the area. Increasing the cohort numbers, multi-centre studies, and optimizing reliability and validity of data, it is most likely that the analysis of EVs will complement the already established clinical parameters and biomarkers in future.