Extracellular vesicles and Wnt signaling: New implications for cancer research
DOI: https://doi.org/10.47184/tev.2023.01.04Extracellular vesicles (EVs) have emerged as important players in intercellular communication, capable of transferring signaling molecules between cells and altering their behavior and function. More and more studies reveal an association of factors of the Wnt pathway with EVs. A tight and coordinated regulation of Wnt signaling is essential for maintaining tissue homeostasis, and dysregulation has been associated with several diseases, including cancer. The influence of Wnt signaling on EV biogenesis has revealed a new mechanism by which Wnt proteins can regulate the creation of a favorable tumor microenvironment promoting the growth and survival of cancer cells. This review summarizes our current understanding of the connections between EVs and Wnt signaling and discusses their relevance in human cancer.
Keywords: extracellular vesicles, Wnt signaling, cancer, biogenesis, biomarkers
Extracellular vesicles
Extracellular vesicles (EVs) are a heterogeneous group of small, membrane-enclosed nanoparticles released by cells into the extracellular space. They can be categorized into two main subpopulations, small EVs (sEVs) and large EVs (lEVs), which differ in their biogenesis, size, and cargo (Fig. 1).
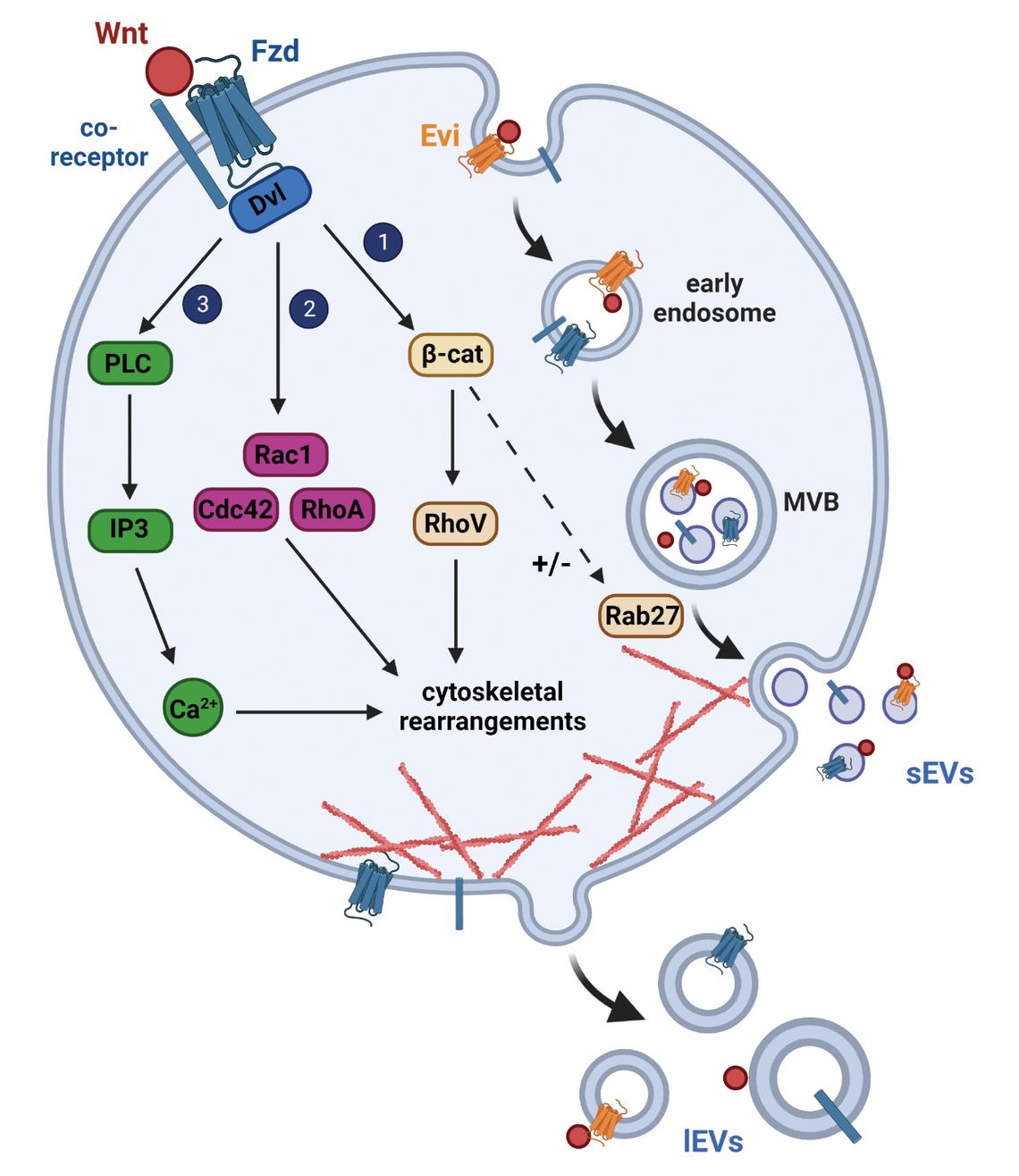
Figure 1: The cellular interplay of Wnt signaling and EV biogenesis. Binding of a Wnt ligand to a Fzd receptor and Wnt co-receptor (e.g., Lrp5/6, PTK7, Ror1/2) recruits Dvl which can relay the Wnt signal via three distinct routes: 1) Mobilization of Wnt/β-catenin signaling which results in RhoV activation and/or regulation (context-dependent activation or inhibition) of Rab27 expression, an important factor for MVB trafficking; 2) Activation of small GTPases, or their GEFs via Wnt/PCP signaling; 3) Increase in intracellular Ca2+ levels via Wnt/Ca2+ signaling. All three pathways converge in cytoskeletal rearrangements and can thus regulate EV formation. Wnt proteins themselves can end up as EV cargo molecules on sEVs and lEVs through their interaction with the Wnt transporter molecule Evi/Wls. How and where Wnt proteins are separated from Evi is still unclear. This figure was created with biorender.com.
In general, the term sEVs describes a heterogeneous mix of particles smaller than 200 nm that are likely to be derived from different cellular compartments. Although at the moment, their distinct origins remains a matter of extensive research and debate, the molecular mechanisms underlying the biogenesis of the sEV population derived from the endosomal system have started to be resolved and have been comprehensively reviewed in [1]. Briefly, sEV biogenesis begins with the invagination of the plasma membrane into the cell, inducing the formation of early endosomes. The budding of late endosomal membranes creates multivesicular bodies (MVBs), containing small intraluminal vesicles (ILVs). The sorting of cargo onto ILVs is mainly mediated by proteins of the endosomal sorting complex required for transport (ESCRT), although also ESCRT-independent mechanisms are known to affect sEV biogenesis [2, 3]. A particular role is attributed to the adaptor protein Syntenin which directly binds proteoglycans of the Syndecan family as well as the ESCRT accessory protein Alix (ALG-2-interacting protein X) and regulates ILV budding and sEV biogenesis [4]. MVBs can finally either fuse with the lysosome, or autophagosome, resulting in ILV degradation, or fuse with the plasma membrane, leading to the release of ILVs as sEVs into the extracellular space. The transport, docking and fusion of MVBs with the plasma membrane critically depend on the cytoskeleton as well as on Rab GTPases, in particular Rab27, Rab35 and Rab11 [5].
In contrast, lEVs are larger than 200 nm and commonly formed by outward budding of the plasma membrane. During cellular activation, changes in intracellular Ca2+ levels result in the activation of Ca2+-dependent proteases (e.g. Calpain) which disrupt membrane-cytoskeletal anchorage and thereby trigger the formation of membrane blebs [6]. Small GTPases, including e.g., Arf6 (ADP-ribosylation factor 6), RhoA (Ras-homologue gene family member A), or Rac (Ras-related C3 botulinum toxin substrate 1) also play important roles in lEV biogenesis. Arf6 induces extracellular signal-regulated kinase (ERK) activation, which is necessary for vesicle shedding [7] and activates Rho signaling pathways. RhoA and its downstream effectors Rock1 and Rock2 (Rho-associated coiled-coil-containing protein kinase 1/2) are crucial regulators of actin dynamics [8]. Finally, proteins of the ESCRT system are thought to play a crucial role in lEV cargo selection and inducing the scission of the vesicle neck, leading to the release of lEVs into the extracellular space [9].
EVs have been found in various body fluids [10] and are being investigated as potential biomarkers for a range of diseases, including cancer (reviewed in [11]). In cancer biology, EVs play a critical role in intercellular communication by carrying tumor factors and signaling molecules to recipient cells [10]. Among the EV cargo molecules, several cytosolic / nuclear (e.g. β-catenin) or membrane (e.g. Ror1) components of the Wnt pathway have been detected. Wnt signaling is involved in various cellular processes and is essential for the development and homeostasis of tissues. In cancer, Wnt signaling is often aberrantly activated and contributes to disease progression [12]. In this review, we provide an overview of the association between EVs and the Wnt signaling pathway, exploring how Wnt proteins regulate EV biogenesis and how Wnt-carrying EVs can shape a favorable tumor microenvironment for promoting cancer cell growth.
Wnt signaling
The 19 identified Wnt proteins are highly conserved cysteine-rich lipoglycoproteins that can interact with the ten members of the Fzd (Frizzled) family and their associated co-receptors (e.g., Lrp5/6, Ror1/2) [13]. The activated downstream Wnt signaling response is classically divided into the β-catenin-dependent (canonical) and β-catenin-independent (non-canonical) pathway.
Canonical Wnt signaling is centered around β-catenin as the key component. In the absence of a Wnt ligand, this unstable protein is bound and phosphorylated in a destruction complex consisting of Axin, APC (adenomatous polyposis coli), CK1α (casein kinase 1α) and GSK3α/β (glycogen synthase kinase 3α/β). The phosphorylation of β-catenin induces its poly-ubiquitination and thus targets it for proteasomal degradation [13]. Upon binding of a canonical Wnt ligand (e.g., Wnt1, Wnt3a) to a Fzd receptor and the LRP5/6 (low-density lipoprotein receptor-related protein 5/6) co-receptor, β-catenin is released from the destruction complex and translocates into the nucleus [12]. Here, it mainly interacts with transcription factors of the T-cell factor/lymphoid enhancer factor (TCF/LEF) family and initiates the transcription of numerous target genes implicated in cell proliferation, survival and stemness [14]. Alternatively, β-catenin can also interact with e.g. MyoD, SOX17 or OCT4 to initiate the transcription of muscle, endoderm or pluripotency self-renewal genes [15].
Non-canonical Wnt signaling, in contrast, is stimulated by the binding of a non-canonical Wnt ligand (e.g., Wnt4, Wnt5a, Wnt5b, or Wnt11) to a Fzd receptor and non-canonical co-receptor such as Ror1/2 (receptor tyrosine kinase-like orphan receptor 1 or 2), Ptk7 (protein tyrosine kinase 7), or Ryk (receptor-like tyrosine kinase) [16, 17]. Comparable to canonical Wnt signaling, binding of a Wnt ligand to its cognate receptors induces the recruitment and activation of DVL. On the one hand, DVL can activate the small GTPases RhoA and Rac1. These, in turn, can initiate Rock1/2, Dia1 (diaphanous-related formin 1) and Jnk (Jun-N-terminal Kinase) which regulate cell motility and polarity through rearrangements in the actin cytoskeleton (Wnt/Planar cell polarity (PCP) pathway) [16, 18]. On the other hand, DVL can activate PLC (phospholipase C) which cleaves PIP2 (phosphatidylinositol-4,5-bisphosphate) to DAG (diacylglycerol) and IP3 (inositol-1,4,5-trisphosphate) (Wnt/Ca2+ pathway) [19]. The secondary messenger IP3 causes an increase in intracellular Ca2+ levels, thereby activating several Ca2+-sensitive kinases and phosphatases which triggers actin polymerization and promote the expression of genes that promote cell growth, survival, invasion, or angiogenesis [19]. Thus, many different potential downstream pathways can be initiated by Wnt receptor activation. The combination of available ligand, the (co-)receptor status of the target cell as well as the presence of certain downstream effectors is thought to govern subpathway activation and functional output of Wnt signaling [20].
Wnt components on EVs
Since the original discovery of active Wnt proteins on sEVs in 2012 [21] and lEVs in 2013 [22], a growing number of studies has identified components of the Wnt signaling pathway as cargo of EVs and revealed their implications for cancer progression. A comprehensive overview of these findings is given in Table 1.
Table 1: Wnt components on EVs.
Factor | Source | EV type | Function | Ref. |
---|---|---|---|---|
Wnt1 | colorectal cancer cells | sEV | promotes colorectal cancer cell proliferation and migration | [23] |
Wnt2b | cervical cancer cells cervical cancer patients | sEV | activates fibroblasts to promote cervical cancer progression | [32] |
Wnt3a | lymphoma cells
lung cancer cells | sEV
sEV | modulates population equilibrium in tumor progression of diffuse large B-cell lymphoma promotes lung cancer progression | [35, 36] |
Wnt4 | colorectal cancer cells
colorectal cancer cells | sEV
sEV | induces cell proliferation and migration of endothelial cells promoting tumor growth and angiogenesis of colorectal cancer enhances migration and invasion of colorectal cancer cells | [24, 25] |
Wnt5a | macrophages
gastric cancer cells gastric cancer patients | lEV sEV sEV | macrophage-induced invasion of breast cancer cells
education of bone marrow-derived mesenchymal stem cells, enhancing gastric cancer migration and invasion | [22, 33] |
Wnt5b | colorectal cancer cells pancreatic cancer cells | sEV | promotes lung cancer cell migration and proliferation | [26] |
Wnt7a | breast cancer cells | sEV | promotes lung metastasis of high metastatic breast cancer cells | [37] |
Wnt7b | cervical cancer cells cervical cancer patients | sEV | more proliferative and proangiogenic behaviors of epithelial cells, mediating cervical cancer tumor growth and angiogenesis | [31] |
Wnt10b | fibroblasts | sEV | promote breast cancer epithelial cell proliferation and metastasis | [38] |
Wnt11 | breast cancer cells | sEV | promotes cancer activity and motility | [39] |
Fzd10 | colorectal cancer cells colorectal cancer patients gastric cancer cells gastric cancer patients hepatic cancer cells cholangiocarcinoma cells | sEV | promotes cancer cell proliferation promotes cancer cell survival and proliferation induces epithelial–to-mesenchymal transition of normal colon epithelial cells prognostic biomarker for colorectal and gastric cancer | [27, 34, 40] |
Ror1 | healthy donors leukemia patients | sEV | the number of Ror1-carrying EVs correlated with the number of leukemic cells | [28] |
β-catenin | kidney cancer cells colorectal cancer cells | sEV | enhances cell motility activates the Wnt signaling pathway in favor of tumor progression in colon cancer cells | [29, 30] |
Of note, the studies have so far almost exclusively focused on sEVs. Whether the same functional effects of EV-associated Wnt factors apply to other EV subpopulations, or are specific for sEVs, remains unknown so far.
In particular, Wnt-associated proteins have been detected on EVs derived from colorectal cancer cells, a tumor entity known for its aberrant activation of Wnt signaling. The proteins include Wnt ligands, such as Wnt1 [23], Wnt4 [24, 25] and Wnt5b [26], Wnt receptors, such as Fzd10 [27] and Ror1 [28], as well as Wnt effectors, like β-catenin [29, 30]. Importantly, the presence of Wnt proteins is not restricted to colorectal cancer, but has also been observed in other tumor types. In 2020, Qiu et al. detected a significant increase in the levels of Wnt7b-positive sEVs in the serum of cervical cancer patients compared to healthy controls and demonstrated that elevated levels correlated with more aggressive disease and shorter patient survival [31]. Other studies found high levels of different Wnt-associated proteins on sEVs, particularly in late-stage patients, such as Wnt2b in cervical cancer [32], Wnt5a in gastric cancer [33], or Fzd10 in colorectal and gastric cancer [34]. Although the number of patients recruited in these studies is quite low and the origin of the Wnt factor-carrying EVs remains currently unknown, these findings emphasize their potential as biomarkers for disease detection, prognosis evaluation and treatment monitoring in liquid biopsy samples of cancer patients.
In order to understand the mechanism how Wnt-carrying EVs can interact with their target cells, it is of immense interest, whether the Wnt proteins are located on the EV surface, and therefore available for interaction with their cognate Wnt receptors on recipient cells, or whether they are enclosed within the vesicles. At present, ultrastructural analyses suggest an expression of the Wnt proteins on the outer EV membrane [21, 22, 35, 39]. Gross and colleagues showed that the Wnt transporter molecule Wls/Evi (Evenness interrupted) is important for shuttling bound Wnt proteins into MVBs, thereby enabling their secretion and expression on sEVs [21]. Interestingly, our own results have revealed that the knockdown of Evi reduced Wnt5a expression not only on sEVs, but also on lEVs [22]. Although further research is warranted to specify the molecular mechanisms of Wnt secretion onto EVs, these results suggest that the Wnt lipoglycoproteins are, at least partially, specifically sorted onto EVs.
The functional impact of Wnt proteins in the tumor microenvironment
Several studies have reported tumor-derived EVs carrying Wnt proteins to promote an aggressive phenotype in surrounding cancer cells. Wnt1 on colorectal cancer-derived EVs was shown to promote the proliferation and migration of the corresponding tumor cell lines [23]. Wnt5b on sEVs from pancreatic or colorectal cancer cells stimulated tumor invasion and migration [26]. Huang et al showed that hypoxia, a common feature of the tumor microenvironment, increased the levels of Wnt4a mRNA in sEVs from colorectal cancer cells [25]. The authors furthermore observed that these sEVs triggered the production of Wnt4 protein in normoxic colorectal cancer cells and enhanced tumor invasion and migration, an effect that was found to depend on the activation of canonical Wnt signaling in recipient cells [25]. A pro-metastatic phenotype of Wnt-carrying EVs has not only been observed in vitro, but also in vivo. In murine 4T1 breast cancer cells, a low metastatic tumor subclone secreted Wnt7a-enriched sEVs, which activated PI3K/Akt/mTOR signaling in highly metastatic tumor cell subclones, increasing their ability to metastasize to the lung upon injection into BALB/c mice [37]. Lung cancer cells exposed to particulate matter (PM2.5), an air pollutant associated with lung cancer incidence and mortality, showed increased expression of Wnt3a on their sEVs, which had a stimulatory effect on the proliferation of lung cancer cells, both in vitro and in vivo [36]. Taken together, these studies indicate that Wnt proteins contribute to the pro-metastatic phenotype of cancer cell-derived EVs by directly stimulating tumor cell proliferation, migration and invasion.
Another possibility, how EV-delivered Wnt factors can support tumor progression, is by modulating the surrounding cells of the tumor microenvironment. Fibroblasts, the most abundant cell type in the stroma, are known to promote cancer cell proliferation, migration, and invasion by secreting extracellular matrix-degrading enzymes, cytokines and growth factors [41]. Liang and colleagues detected elevated levels of Wnt2b in sEVs from cervical cancer cells, which induced the transformation of stromal fibroblasts into cancer-associated fibroblasts to promote cancer progression via canonical Wnt signaling [32]. Similarly, the transfer of Wnt7b mRNA in sEVs secreted from cervical cancer cells induced proliferation and angiogenesis in endothelial cells by activating canonical Wnt signaling [31]. Our own results have highlighted the role of Wnt5a in the reprogramming of tumor-associated macrophages. We observed breast cancer-derived lEVs as well as sEVs to induce Wnt5a expression in macrophages, which shuttled the protein on their own EVs back to the tumor cells to induce cancer cell invasion [22]. Another example for Wnt proteins on stromal cell-derived vesicles is Wnt10b, which was transported on fibroblast-derived sEVs to breast cancer cells and activated canonical Wnt signaling, resulting in epithelial-to-mesenchymal transition and increased metastasis formation [38]. A similar misuse of fibroblast-derived EVs for tumor progression has also been reported for Wnt11. Luga and colleagues found that sEVs secreted by fibroblasts were internalized by breast cancer cells and upon trafficking, were loaded with the non-canonical Wnt protein Wnt11 [39]. Wnt11-loaded sEVs then stimulated Wnt/PCP signaling in surrounding malignant cells, probably through interaction with Fzd6, and thus enhanced breast cancer cell motility and metastasis [39]. These studies highlight the involvement of Wnt proteins in reciprocal intercellular communication loops in the tumor stroma and their pivotal role for shaping a pro-tumoral microenvironment. Still, only few studies have thoroughly investigated the mechanisms underlying the function of Wnt-carrying EVs, in particular whether the effects depend on the uptake of the EV-delivered Wnt proteins, or whether dynamic extracellular receptor-ligand interactions are involved for activating downstream signaling. Some studies have shown that EVs carrying Wnt7b mRNA, Wnt4a protein or the Fzd10 receptor, require uptake, and thus cargo delivery, into the target cells for the functional effects [25, 31, 40]. Of note, a recent report described that mutant β-catenin secreted from colorectal cancer cells in sEVs was detectable in the nucleus of neighboring tumor cells and able to trigger transcription of canonical Wnt target genes [30]. As mutant β catenin is constitutively active, its transfer potentially not only activates Wnt signaling in recipient cells, but also other Wnt-related pathways such as e.g. PI3K/Akt/mTOR or TGF-β. Considering that endocytosis is regarded as the main mechanism for EV uptake [1], this observation suggests an endosomal escape mechanism for EV-enclosed (Wnt) cargo proteins, although its mode of action remains elusive at present.
The influence of Wnt signaling on EV biogenesis
In the last decade, it became apparent that through their large-scale regulation of subcellular signaling cascades, Wnt proteins are not just EV cargo molecules, but are actively involved in the regulation of EV biogenesis (Fig. 1). Ekström and colleagues showed that the non-canonical Wnt ligand Wnt5a induces the secretion of sEVs from melanoma cells in an auto- and paracrine manner [42]. They demonstrated that sEV release is not due to transcriptional induction of Wnt5a target genes, but because of the activation of non-canonical Wnt/Ca2+ signaling. Indeed, Wnt5a was shown to induce an increase in intracellular Ca2+ causing a change in F-actin organization and increased sEV secretion which was dependent on the small actin-regulating RhoGTPases Cdc42 (cell division cycle 42), and to a lesser extent, Rac1 [42]. Of note, the tumor sEVs secreted upon Wnt5a stimulation contained immunomodulatory factors, such as Interleukin-6 (IL-6), as well as pro-angiogenic factors, such as vascular endothelial growth factor (VEGF) and matrix metalloproteinase-2 (MMP2) which all are important mediators for creating a tumor-supporting microenvironment. Next to Cdc42 and Rac1, other small GTPases have been identified as important regulators of EV formation. Among them, in particular the known non-canonical Wnt downstream target RhoA as well as its activator ARHGEF18 (Rho/Rac guanine nucleotide exchange factor 18) [43] that have been described to stimulate EV release [8, 44, 45]. RhoV (Ras homolog family member V), which can be activated by canonical Wnt signals [46], has equally been identified as an important activator of sEV secretion [45]. Given the involvement of cytoskeletal rearrangements and actomyosin contractility in lEV budding from the plasma membrane and endosomal transport, these findings are not surprising.
EV secretion can also be directly regulated by canonical Wnt signaling. One example is the degradation complex component APC which is mutated in about 50 % of colorectal cancer patients, leading to a permanent activation of the canonical Wnt pathway. In colorectal cancer organoids, the introduction of an APC mutation was linked to increased secretion of sEVs [47], suggesting that β-catenin plays a central role in regulating sEV release. This is supported by data from colon cancer stem cells in which the ectopic expression of β-catenin stimulated β-catenin/TCF-mediated expression of Rab27b, which is critical for the transport of MVBs to the plasma membrane, and thus stimulated the release of sEVs [48]. In contrast, in leukemic K562 cells activation of the canonical Wnt pathway by Wnt3a stimulation, or GSK3 inhibition, resulted in a decrease in sEV secretion which was explained by a transcriptional downregulation of Rab27 and thus decrease in sEV secretion [45]. Therefore, the Wnt-mediated regulation of Rab27 expression seems to be highly context-dependent as also suggested by the observations of Lu and colleagues [45]. A huge genome-wide screen for regulators of EV biogenesis furthermore identified the Wnt signaling activators LYPD6B (LY6/PLAUR domain containing 6B) and RNF146 (ring finger protein 146) to support sEV secretion, while the negative Wnt regulator NKD2 (NKD inhibitor of Wnt signaling pathway 2) inhibited sEV biogenesis [45]. The regulation of sEV secretion by Wnt signaling was recently confirmed in a more physiological context in patient-derived organoids in which Wnt activation was linked to increased cell proliferation and sEV release [49]. This observation was made in normal as well as cancer-derived organoids, suggesting that the described mechanism is not disease-specific [49].
While mechanistic studies addressing the role of Wnt signaling in the context of EV biogenesis are still scarce, several studies have found links between Wnt signaling and the Src tyrosine kinase [50] as well as ESCRT proteins, including e.g. Tsg101 (tumor susceptibility gene 101) [26], Vps4 (vacuolar protein sorting 4) [51], or Chmp4B/C (charged multivesicular body protein 4B/C) [52], which are all important regulators of EV biogenesis [4, 53]. Therefore, the critical role of Wnt signaling in the regulation of EV secretion is likely to be cemented in the future.
Conclusion
Aberrant activation of Wnt signaling is frequently observed in human cancer. Traditionally, Wnt proteins have been regarded as secreted lipoglycoproteins, until EVs were identified as carriers of active Wnt proteins. The association with EVs is thought to enhance the long-distance signaling capacity of the hydrophobic Wnts in the aqueous extracellular space [54] and can thus potentially support the distribution of Wnts within the organism. The presence of Wnt-positive EVs in human plasma samples highlights their potential as circulating biomarkers for various cancer types with even new avenues for therapeutic interventions. Currently, two modes of action emerge, how the Wnt pathway interacts with EVs to support cancer progression. On the one hand, Wnt signals regulate factors that control the secretion of EVs and thereby actively shape intercellular communication within the tumor microenvironment. On the other hand, Wnt-carrying EVs have emerged as central players in the reprogramming of tumor-adjacent stroma cells, creating a niche that favors tumor progression. A deeper understanding of the underlying molecular mechanisms will allow to develop strategies to specifically target these two processes for cancer treatment in the future.
Acknowledgements
This project was supported by the Deutsche Forschungsgemeinschaft (DFG, German Research Foundation - project 424252458) and the Else Kröner-Fresenius-Stiftung (project 2019_A162).