Extracellular vesicles in transkingdom communication
Extracellular vesicles in plant host-microbe interaction
DOI: https://doi.org/10.47184/tev.2019.01.07Recent pioneering works resulted in novel concepts that place EVs carrying regulatory small RNAs as central players in inter-species and cross-kingdom communication with emphasis on host-pathogen, host-parasite and host-microbiome interactions.
Keywords: Extracellular vesicles, outermembrane vesicles, bacteria, fungi, plants, inter-species, cross-kingdom, small RNAs
Introduction
In 2015, a satellite workshop at the International Symposium on Extracellular Vesicles (ISEV) in Sao Paulo, Brazil, emphasized on the potential significance of EVs in cross-kingdom communication in hosts, microbes and parasites (1). As an important conclusion of this meeting, the necessity to develop robust protocols for EV isolation and the identification of suitable biomarkers for the different types of EVs was pointed out, in order to advance the study of specific roles of EVs in host-pathogen interactions. In August 2018, around 50 scientists from all over the world came together for a mini-symposium on EVs in inter-organismal communication in Munich, at the Ludwig-Maximilians University. The scientific discussions included new findings of EVs discovered in plant, fungal, oomycete, nematode and bacterial systems, as well as exchange of current opinions on how to integrate such discoveries into the current concepts of EVs in inter-species and cross-kingdom communication (2). As two important goals for the future, the participants defined a better understanding of EV biology across kingdoms, and the extension of our knowledge on how EVs participate in plant immunity and in plant-pathogen interaction.
Plants recognise by pattern recognition receptors (PRRs) pathogen molecular patterns located at the cell periphery, which activate cellular immune signalling. Host-adapted plant pathogens secrete small peptides, called effectors that interact with, and sometimes enter, plant cells to suppress host immunity. In defence, plants evolve nucleotide-binding domain leucine-rich repeat containing (NLR) receptors that perceive pathogen effectors and mount an effector-triggered immunity. To escape recognition, pathogens co-evolve their effector repertoires, leading to a continual molecular arms race between pathogens and host plants (3). During plant-pathogen interactions, pathogen effectors and plant antimicrobial molecules must be transported through the extracellular space between plant and pathogen cells, without getting degraded or diluted, to be functional. Extracellular vesicles (EVs) fit perfectly to take over this function (Figure 1).
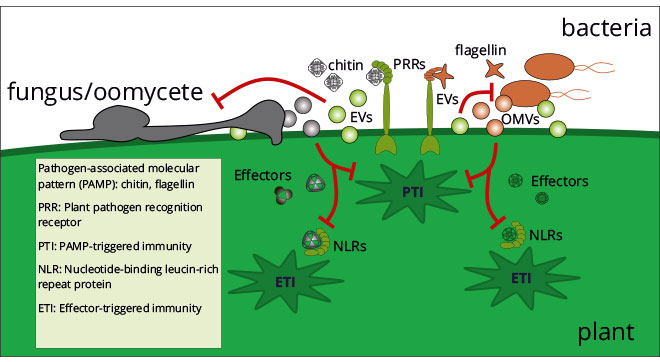
Indeed, EVs mediate intra-organismal cell-to-cell communication in mammals with impact on disease progression, cancer development as well as on immune activation (4). Important to note, the biogenesis of EVs is conserved over the entire tree of life including Archaea, bacteria, fungi, oomycetes, protozoa, animals, and plants. And this fact clearly shows on the one hand the ubiquitous prevalence of EVs in biology, and on the other hand the possibility that EVs serve as communication channel between hosts and microbes with beneficial or pathogenic outcomes (5-8). Here, we discuss the state-of-the-art regarding potential roles of EVs for host-pathogen interaction by highlighting significant achievements and by summarizing recent discussions on conceptual open questions, for instance: Where do EVs come from? What molecules do EVs transport? How are cargo molecules loaded into EVs? How do EVs pass across cellular barriers and extracellular matrices? What is the biological function of EVs in host-pathogen interactions?
Where do EVs come from?
EVs are lipid bilayer particles that are synthesized and released by cells via unconventional secretion pathways (9). In mammals, exosome-like EVs derive from intracellular multivesicular bodies (MVB) that fuse to the cytoplasm membrane and release EVs into the periplasm and the extracellular lumen. A recent study also links EVs of the model plant Arabidopsis thaliana to MVBs (10), indicating that biogenesis of EVs seems to be well conserved in plants and animals. Moreover, tetraspanins that are EV transmembrane spanning proteins in mammalian exosome-like EVs are also detected in Arabidopsis EVs (10), suggesting that plant EVs are true exosomes. Another study reported on the syntaxin PEN1 being enriched in Arabidopsis EVs. PEN1 is a known factor of intracellular membrane trafficking and endo/exocytosis, and has been described to participate in plant immune response (11).
In microbes, biogenesis of EVs is inadequately understood. Fungi and oomycetes form MVBs, suggesting that exosome-like EVs may also exist in eukaryote microbes (12). Since bacteria do not possess endomembrane organelles, EV release is considered to appear via outer-membrane budding, thus EVs are often called outer-membrane vesicles (OMVs) (13). Genetic manipulation strategies (e.g. gene knockout) in microbes have not delivered any EV null mutant strain, which could genetically link secretion pathways to EV biogenesis and release, but mutants only exhibited moderate, quantitative differences in EV number or size (14, 15). In fact, EV populations are rather heterogeneous in size, concentration and cargo composition indicating that different secretion pathways of EVs might be in place. And this offers an explanation why a single gene mutation does not consequently lead to a “zero EV” phenotype. To deepen our understanding of EV biogenesis and its connection to the secretory pathways, attempts that combine genetics with biochemical inhibitors that specifically block certain steps in a secretion process are definitely worth trying.
What is transported in EVs?
Over the last years, mass spectrometry and high-throughput sequencing analyses allowed identification of several proteins and nucleic acids, mainly messenger RNAs (mRNAs) and non-coding small RNAs (sRNAs), as EV cargoes. For instance, comparative analysis of EV proteomes from different fungal species revealed common sets of molecular function or biological process, such as cell wall modelling, indicating a conserved role of EVs in fungi (16). Frequently, known virulence-associated proteins were described in EVs isolated from pathogenic bacteria and fungi, suggesting EVs as translocation vehicles for virulence factors towards the host (16, 17). Moreover, antimicrobial proteins have been determined in plant EVs upon bacterial infection (11). Beside proteins, diverse RNA species in bacterial, fungal and plant EVs and have been detected and analysed by next generation sequencing. In particular, gene-regulatory RNAs were discovered and are suggested to mediate gene regulation in cells and organisms that incorporate EV-RNAs during host-pathogen interaction (11, 18). Other types of RNAs were also discovered in EVs, such as mRNAs or long non-coding RNAs that might be functional in host-microbe communication, too. Nevertheless, the importance of EV proteins and RNAs in host-pathogen interactions needs to be explored in greater detail.
EVs in host-pathogen interactions
To test the function of pathogen EVs for infection, host cells and tissues are often treated with pathogen EV extracts in culture. Fluorescence labelling of EVs may allow visualization of EV-uptake by host cells or tissues. Several immunomodulatory effects were found in different mammalian host cell types upon pathogen EV treatment. For instance, EVs of human pathogenic fungi and bacteria can stimulate or repress host immunity by redirecting immunity-related factors, such as cytokines, in macrophages and other immunity-related cell types (5, 6). These examples indicate that pathogens use EVs as virulence factors, while host cells have evolved recognition of pathogen EVs for immune activation. However, from the basic research point of view, in-vitro culture assays provide only limited insights into how important EVs are during host-pathogen interaction at the organismal level. To further address this open question, EVs should be studied directly at the host-pathogen interface, with blocking their production or activity during infection to reveal their real biological significance.
EV-mediated delivery of small RNAs (sRNAs) that trigger inter-species or even cross-kingdom RNA interference (ckRNAi) has been documented in animal-parasite and plant-fungal interactions (19). For example, EVs of parasitic nematodes carry conserved microRNAs that mediate type-II immune suppression in mice (20). Moreover, aggressive fungal plant pathogens, such as Botrytis cinerea, deliver sRNAs into host plant cells that hijack the plant RNAi pathway to silence host immunity genes for infection (21). However, whether fungal sRNAs are transported into plants via EVs needs to be demonstrated. This phenomenon of ckRNAi is bi-directional, because plants transfer small interfering RNAs (siRNAs) into attacking fungal pathogens, too, that suppress fungal virulence genes. Hereby, plant siRNAs are transported into infecting pathogens by exosome-like EVs (9). Plant host-induced gene silencing (HIGS) reflects yet another RNAi-based communication between plants and pathogens and is a tool to engineer crop plant resistance against notorious plant pathogens/pests (22). HIGS has been employed even before the discovery of natural ckRNAi, inspired by environmental RNAi found in nematodes (23). How important EVs are for the delivery of plant HIGS RNAs into pathogens/pests is not known, yet. Taken together, these observations suggest that EVs are a central player in inter-species and cross-kingdom RNA communication (Figure 2).
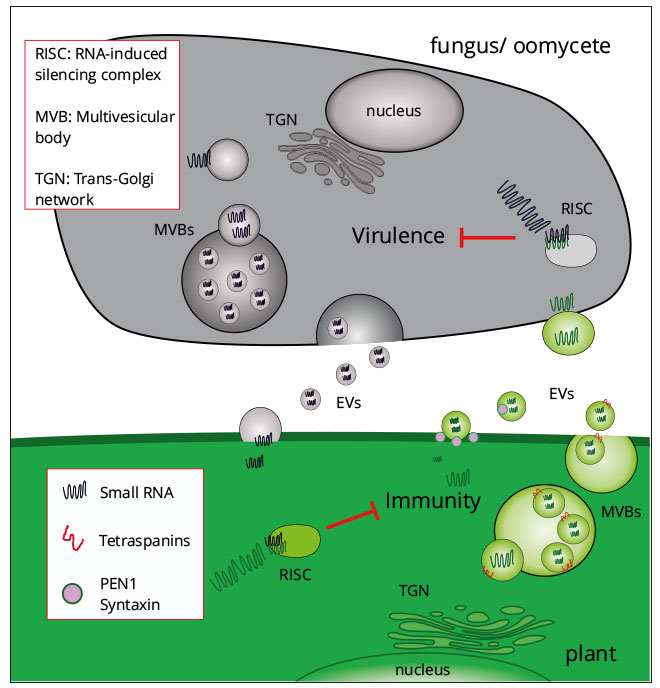
An interesting new direction of EV-mediated communication during infection is reported at the microbial population level. EVs play a functional role in the “division of labour” phenomenon observed in Cryptococcus neoformans var. gattii that is critical to activate infectivity. In this regard, EVs are a communication channel between phagocytosed cryptococcal individuals and the extracellular Cryptococcus population (24). Moreover, recent reports indicate that microbiota-derived vesicles might be protective against diseases in the gut system (25). Furthermore, diet-consumed plant-derived exosome-like EVs (sometimes called dietary EVs) might be taken up by the gut microbiota with effects on the host wellbeing (26). These latest observations open new perspectives onto EVs as pluripotent multi-organismal communication media to direct microbial organisation connected to plant and animal host fitness.
How do EVs pass cellular barriers?
Cellular release of EVs by bacterial, fungal and plant cells raise the question of how do lipid membrane particles of 50-500 nanometres in diameter pass complex cellular barriers, such as the periplasmic matrix and the cell wall. Is the plasticity of the cell wall way higher than formerly expected? The shape and size of EVs might be flexible as well, thus, EVs might fit through cell wall pores and channels despite the EV size (27). This is supported by observations made by scanning EM showing the transfer of AmBisome® particles, a commercial antifungal liposome formulation, through the fungal cell wall (28). EM tomography at the fungal-plant interface revealed multiple morphologies of extracellular lipid formations, from round-shaped individual particles to interconnected tube-like membrane structures and networks (29, 30). Cell wall remodelling enzymes that have been identified as part of the EV cargo in fungi might break down local cell wall structures to facilitate passage of EVs in particular through these tight matrices. Finally, plant pathogens, such as B. cinerea, release cell wall-degrading enzymes during host colonization, which soften the plant cell wall and thus might promote fungal EV entry into plant cells. Other types of plant-interacting microbes can form intimate cell/cell contacts with accommodating host plants, at which cell walls are largely removed, such as the haustorium of pathogens, the arbuscle of plant-mycorrhizal fungi, or the nodule of nitrogen-fixing bacteria. These interfaces enable extensive bi-directional molecular exchanges, possibly through microbial and plant EVs.
Conclusion
EVs attract growing attention in the field of molecular plant-microbe interactions. The transport of pathogenic proteins and sRNAs into host plant cells by EVs offers an answer to a longstanding question regarding the mechanism of effector delivery. Moreover, EVs are presently secreted by all types of living cells, pathogenic or not, and EV-transmitted regulatory RNAs can serve as a unique message understood by all life forms, as it largely relies “only” on nucleobase pairing (18).
In order to advance our understanding in the biological function of EVs in host-microbe interactions, the development of reliable protocols and standards for EV purification, visualization, and identification via proper EV biomarkers as well as the analysis of their molecular composition are required. Only then, investigation of EVs in situ will become a key to decode the communication code of EVs between cells to achieve accommodation, infection, or immunity that will extend our views on EVs in host-microbe interactions.